Longsheng Wang, Junli Wang, Yushan Wu, Yuehui Sun, Songsui Li, Lianshan Yan, Yuncai Wang, Anbang Wang, "Chaos synchronization of semiconductor lasers over 1040-km fiber relay transmission with hybrid amplification," Photonics Res. 11, 953 (2023)

Search by keywords or author
- Photonics Research
- Vol. 11, Issue 6, 953 (2023)
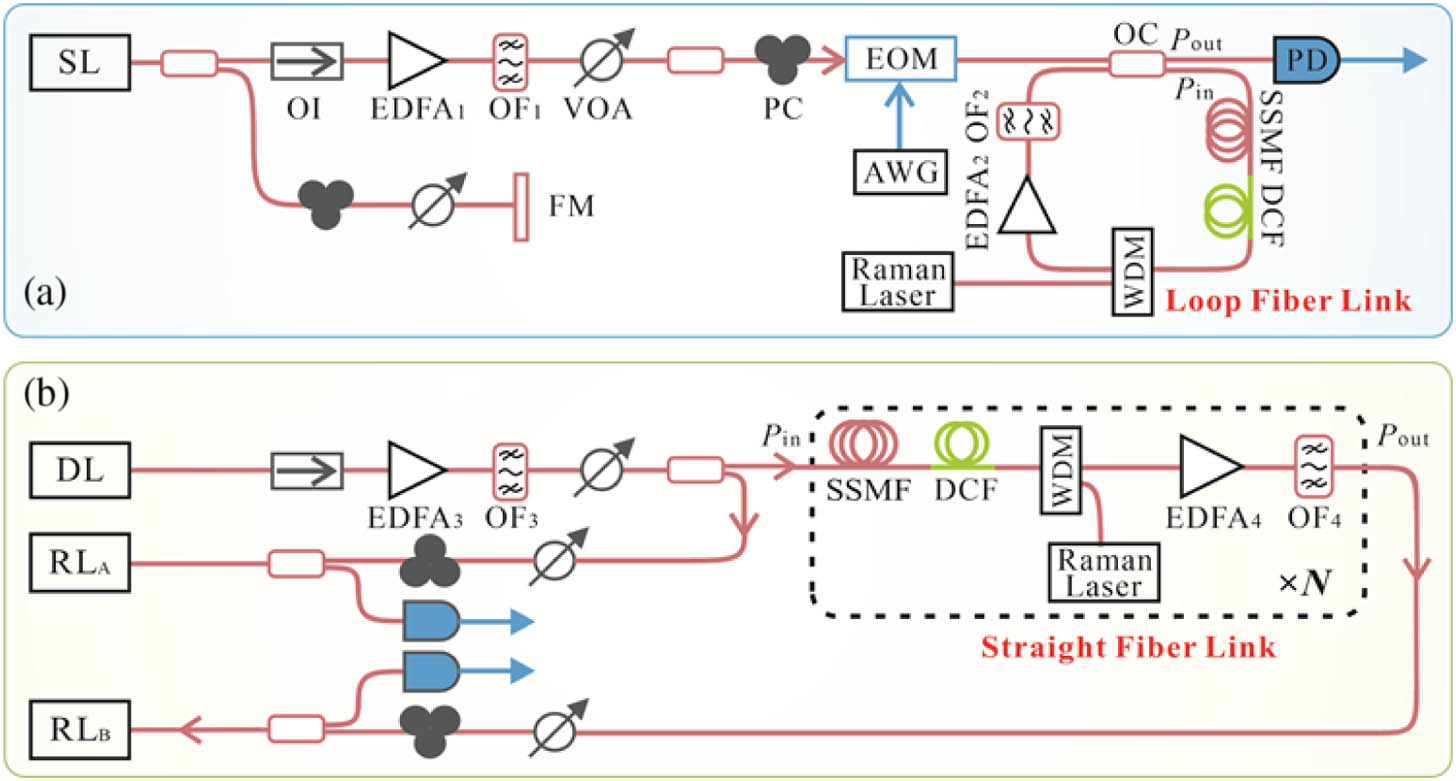
Fig. 1. (a) Fiber-loop experiment for investigating fidelity transmission of laser chaos; (b) setup of long-reach chaos synchronization. SL: semiconductor laser; DL: drive laser; RL A , B : response lasers; OI: optical isolator; EDFA: erbium-doped fiber amplifier; OF: optical filter; VOA: variable optical attenuator; FM: fiber mirror; PC: polarization controller; EOM: electro-optic modulator; AWG: arbitrary waveform generator; OC: optical coupler; SSMF: standard single-mode fiber; DCF: dispersion compensation fiber; WDM: wavelength division multiplexer; PD: photodetector.
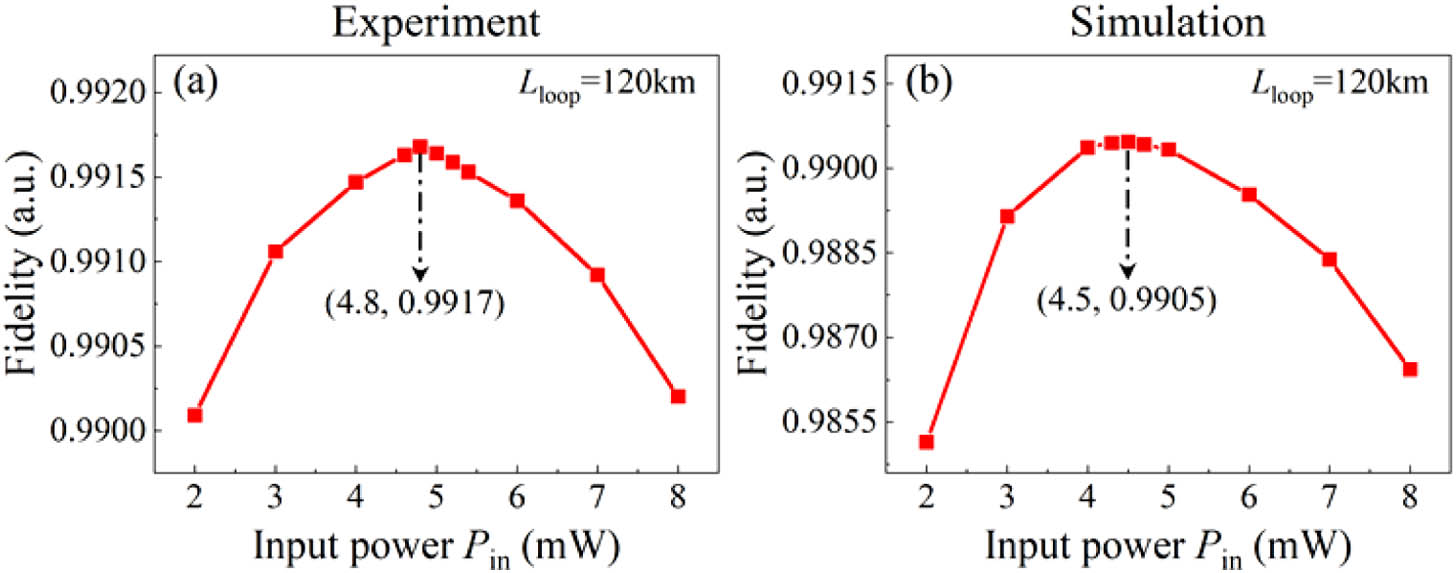
Fig. 2. Single-span fiber transmission. (a) Experimental and (b) simulated fidelities as a function of optical power launched into the fiber for a fixed loop length L loop = 120 km . EDFA gain G E = 34.7 dB .
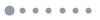
Fig. 3. Single-span fiber transmission. (a) Experimental and (b) simulated maximum fidelities and optimum launching power as a function of fiber loop length.
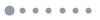
Fig. 4. Multispan fiber transmission with the EDFA relay. (a) Experimental and (b) simulated maximum fidelities as a function of relay number for different fiber loop lengths L loop = 90 km , 100 km, and 120 km.
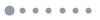
Fig. 5. Single-span fiber transmission. (a) Experimental and (b) simulated fidelity as a function of optical power launched into the fiber at different gain ratios of the DFRA and the EDFA for a fixed fiber loop length L loop = 120 km . Total gain G D + G E = 34.7 dB .
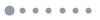
Fig. 6. Single-span fiber transmission. (a) Experimental and (b) simulated maximum fidelity, optimum launching power, and gain ratio as a function of the fiber loop length.
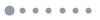
Fig. 7. Multispan fiber transmission with the EDFA and the DFRA relay. (a) Experimental and (b) simulated maximum fidelities as a function of relay number for different fiber loop lengths L loop = 120 km , 130 km, and 150 km.
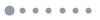
Fig. 8. Experimental results of a 1040-km chaos transmission over a straight fiber link using hybrid amplification. (a) Optical spectra; (b) radio-frequency spectra; (c) temporal waveforms; (d) scatter plot. P in = P out = 5.4 mW , the DFRA gain G D = 7.0 dB , and the EDFA gain G E = 30.0 dB .
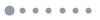
Fig. 9. Experimental results of 1040-km chaos synchronization. (a) Optical spectra; (b) radio-frequency spectra; (c) temporal waveforms; (d) scatter plot. The injection strengths of RL A and RL B are 78% and 57%, respectively.
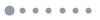
Fig. 10. 1040-km chaos synchronization coefficient as a function of (a) time and (b) chaos bandwidth after low-pass filtering (LPF).
|
Table 1. Simulation Parameters of the Semiconductor Laser
|
Table 2. Simulation Parameters of the Fiber, the Filter, and the Amplifier
|
Table 3. Parameters of Eight-Span Fibers
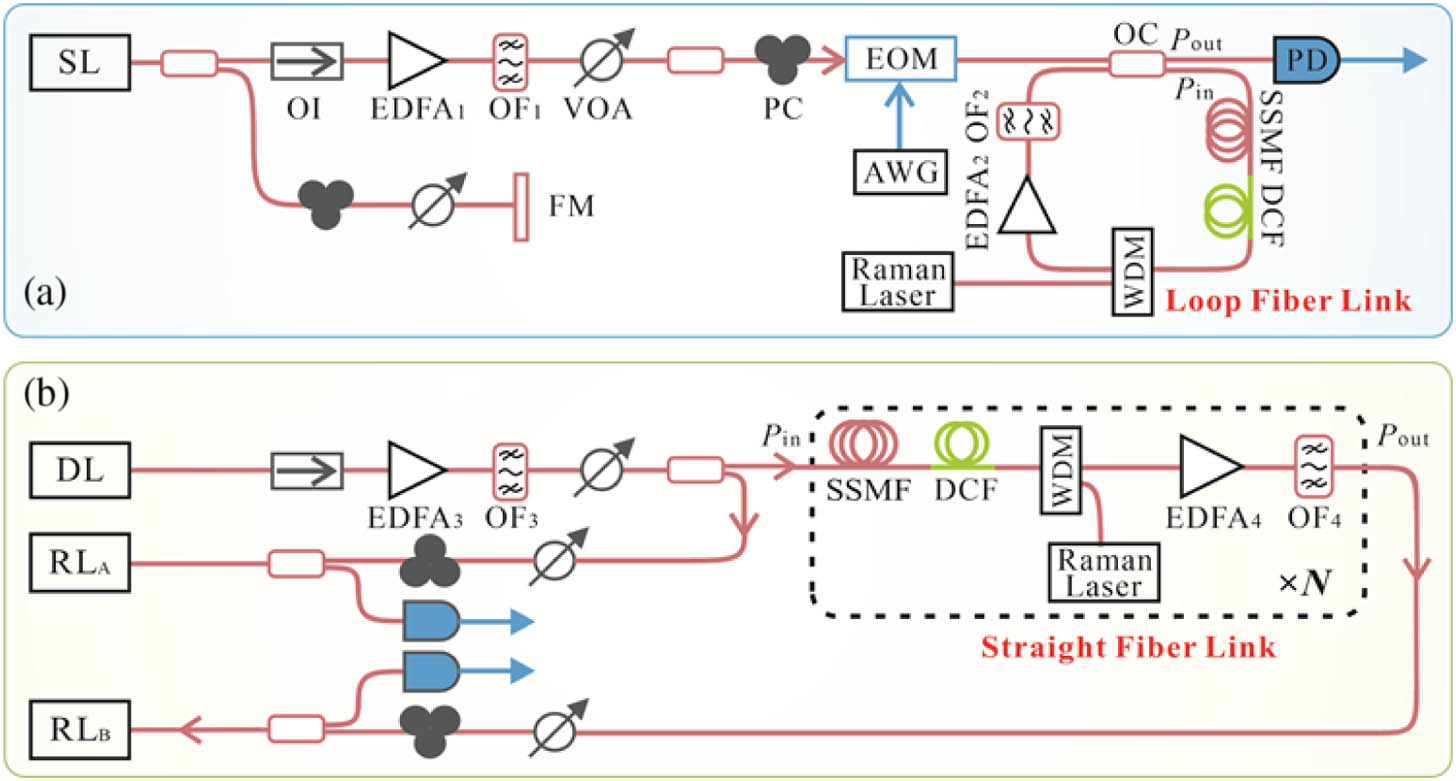
Set citation alerts for the article
Please enter your email address