Author Affiliations
1Southern University of Science and Technology, College of Engineering, UTS-SUSTech Joint Research Centre for Biomedical Materials and Devices, Department of Biomedical Engineering, Shenzhen, China2City University of Hong Kong, Department of Biomedical Engineering, Hong Kong, China3Peking University, College of Future Technology, Department of Biomedical Engineering, Beijing, China4University of Technology Sydney, Institute for Biomedical Materials and Devices (IBMD), Faculty of Science, Sydney, Australiashow less
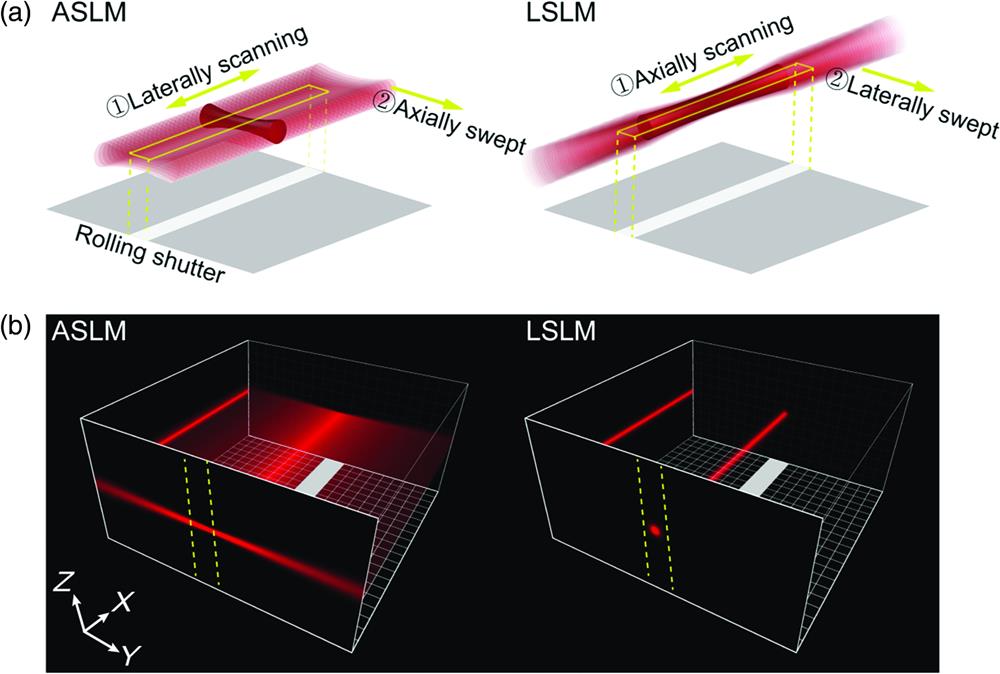
Fig. 1. Schematic comparison between the ASLM and LSLM. (a) In the ASLM, a focused Gaussian beam is first laterally scanned that generates a light sheet perpendicular to the direction of beam propagation. Afterward, the focus of the Gaussian beam is axially swept in synchronization with the rolling shutter of the camera. In the LSLM, a Gaussian beam is first axially scanned that forms a “light needle” along the direction of beam propagation. Then, the beam is laterally swept in synchronization with the camera. Here, the axial direction is along the propagation of the beam and the lateral direction is perpendicular to the propagation of the beam. With the rolling shutter, only the region excited by the in-focus, thin light sheet is imaged by the camera. (b) Comparison of light sheets generated by lateral scanning of the ASLM and axial scanning of the LSLM. The images on the plane show the cross section of the light sheet, and the yellow dashed lines indicate the rolling shutter. With the same rolling shutter, the ASLM shows better axial optical sectioning, but the LSLM contains more excitation power in the shutter region, which is more photon-efficient.
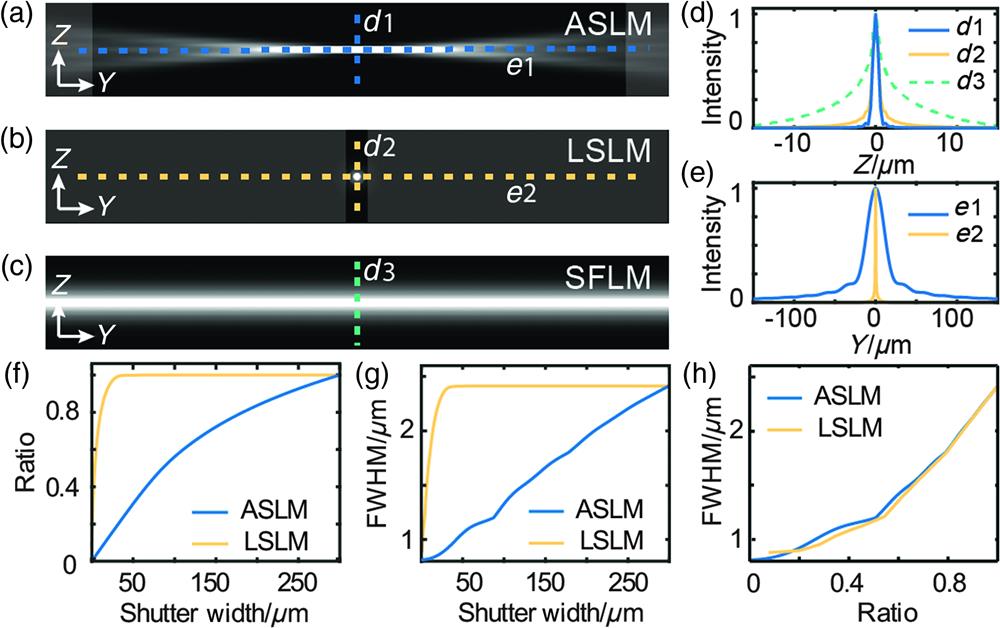
Fig. 2. Comparison between the ASLM and LSLM with simulation studies. Cross-sectional view of the light sheet with a cropped -FoV of in the plane after the first scanning for (a) the ASLM and (b) the LSLM. The highlighted region shows an example of the rolling shutter with a width of for the ASLM and for the LSLM, for which the energy ratios are both and the axial FWHM are both for the two models. Scale bar: . (c) Cross-sectional view of the SFLM in the plane generated by lateral and axial scanning without confocal detection. (d) Intensity profiles along the axis for , , and . The FWHM is for the ASLM, for the LSLM, and for the SFLM. (e) Intensity profiles along the axis for and . (f) The energy ratio changes with rolling shutter widths for both the ASLM and LSLM. (g) The axial FWHM increases with a larger rolling shutter width for both the ASLM and LSLM. (h) Relationship between the axial FWHM and energy ratio.
Fig. 3. Simulated imaging results of fluorescent beads. (a) and (b) Simulated imaging results of fluorescence beads in the and planes, and the three insets show the imaging results of the white dashed areas in the ASLM, iLSLM, and SFLM. (c) The upper and lower parts show the profiles of the ASLM, iLSLM, and SFLM when the photon efficiencies reach 58% and 80%, respectively. (d) and (e) Simulations to compare the photon efficiency and the axial FWHM of the ASLM and iLSLM with increasing rolling shutter. (f) Relationship between photon efficiency and the axial FWHM of the ASLM and iLSLM. Scale bar: .
Fig. 4. The microscopy setup and focus scanning with the SLM. (a) Schematic diagram of the light sheet microscope. Laser: 473 nm, bandwidth 0.2 nm, MBL-III-473, CNI; : achromatic plano-convex lens, , , ; HWP1 and HWP2: half-wavelength plate, WPA2420-450-650, Union Optic; PBS, polarization beam splitter; CCM1-PBS251/M, Thorlabs; SLM, spatial light modulator, QXGA-R11, ForthDD; EO: excitation objective Lens, 20X/N.A. W, Olympus; DO: detection objective Lens, 10X/N.A. W, Olympus; Filter: ET525/50 M, Chroma; TL: tube lens, 200 mm, C60-TUBE B, ASI; Camera: ORCA-Flash 4.0, Hamamatsu. (b) and (c) When a binary phase pattern is loaded onto the SLM, the focus of the beam can be scanned in three dimensions. (d) Corresponding images when different phase patterns are loaded onto the SLM.
Fig. 5. Experimental imaging results of fluorescent beads and U2OS cells. (a) and (b) Experimental imaging results of fluorescence beads in the and planes, and the three insets show the imaging results of the white dashed areas in the ASLM, iLSLM, and SFLM. (c) The upper and lower parts show the profiles of the ASLM, iLSLM, and SFLM when the photon efficiencies reach 58% and 80%, respectively. (d) and (e) The photon efficiency and axial FWHM of the ASLM and iLSLM corresponding to the increasing rolling shutter. (f) Relationship between the photon efficiency and the axial FWHM of the ASLM and the iLSLM. (g)–(i) The ASLM and iLSLM imaging results of U2OS cells corresponding to the photon efficiencies of 53% and 80%. Scale bar: .