Linda Shao, Jin Zhang, Ivan D. Rukhlenko, Weiren Zhu, "Electrically reconfigurable microwave metasurfaces [Invited]," Chin. Opt. Lett. 20, 103601 (2022)

Search by keywords or author
- Chinese Optics Letters
- Vol. 20, Issue 10, 103601 (2022)
![(a) Working principle of voltage-induced nematic LC[98]. (b) Unit cell of electric resonator with LC in a microfluidic channel (light blue) and sealing Pyrex top cover (left) and ELC simulated frequency resonance shift from 4.61 to 4.35 GHz (right)[99]. (c) Tunable LC-based Fabry–Perot resonator, where the gray area is filled with LCs and sandwiched between two external substrates supporting the reflecting mirrors (left) and measured transmission coefficient of the fabricated LC-based Fabry–Perot resonator (right)[100]. (d) Structure of nematic LC tunable filter and its measured S11 parameters for various peak voltages[101]. (e) Tunable short wire-pair type of metamaterial based on nematic LC and its experimental transmission magnitude as a function of the external dc bias voltage[102]. (f) Experimental setup of LC-based coding metamaterial and its beam steering performance at 54 GHz[103].](/richHtml/col/2022/20/10/103601/img_001.jpg)
Fig. 1. (a) Working principle of voltage-induced nematic LC[98]. (b) Unit cell of electric resonator with LC in a microfluidic channel (light blue) and sealing Pyrex top cover (left) and ELC simulated frequency resonance shift from 4.61 to 4.35 GHz (right)[99]. (c) Tunable LC-based Fabry–Perot resonator, where the gray area is filled with LCs and sandwiched between two external substrates supporting the reflecting mirrors (left) and measured transmission coefficient of the fabricated LC-based Fabry–Perot resonator (right)[100]. (d) Structure of nematic LC tunable filter and its measured S11 parameters for various peak voltages[101]. (e) Tunable short wire-pair type of metamaterial based on nematic LC and its experimental transmission magnitude as a function of the external dc bias voltage[102]. (f) Experimental setup of LC-based coding metamaterial and its beam steering performance at 54 GHz[103].
![(a) Multiple transfer-etch processing for a two-layer device[123]. (b) Measured absorption spectra of single graphene-quartz absorbers with 1–4 graphene layers on quartz[123]. (c) Cross-sectional view and photograph of sandwich graphene structure[60]. (d) Sandwich graphene structure-based coherent perfect absorber illustrated by two counter-propagating and coherently modulated input beams (I+ and I−), with O+ and O− being the output beams[59]. (e) Salisbury screen based on sandwich graphene structure and its broadband reflection spectrum for various bias voltages[60]. (f) Five-layer graphene absorber and its reflection spectrum for different bias voltages[124]. (g) Three-dimensional structure and photograph of tunable absorber based on sandwich graphene structure and high impedance surface[103]. (h) EIT analog of graphene-based metasurface and its transmission spectrum for various bias voltage[125]. (i) Optically transparent graphene-based absorbing metasurface and its tunable absorption for different sheet resistance[126]. (j) Three-dimensional structure and reflection spectrum of dual-tunable metasurface based on a combination of graphene and active resonators[127].](/richHtml/col/2022/20/10/103601/img_002.jpg)
Fig. 2. (a) Multiple transfer-etch processing for a two-layer device[123]. (b) Measured absorption spectra of single graphene-quartz absorbers with 1–4 graphene layers on quartz[123]. (c) Cross-sectional view and photograph of sandwich graphene structure[60]. (d) Sandwich graphene structure-based coherent perfect absorber illustrated by two counter-propagating and coherently modulated input beams (I+ and I−), with O+ and O− being the output beams[59]. (e) Salisbury screen based on sandwich graphene structure and its broadband reflection spectrum for various bias voltages[60]. (f) Five-layer graphene absorber and its reflection spectrum for different bias voltages[124]. (g) Three-dimensional structure and photograph of tunable absorber based on sandwich graphene structure and high impedance surface[103]. (h) EIT analog of graphene-based metasurface and its transmission spectrum for various bias voltage[125]. (i) Optically transparent graphene-based absorbing metasurface and its tunable absorption for different sheet resistance[126]. (j) Three-dimensional structure and reflection spectrum of dual-tunable metasurface based on a combination of graphene and active resonators[127].
![Reconfigurable metasurfaces with active lumped elements. (a) Design of switchable microwave absorber[133]. (b) Single active metasurface to achieve reconfigurable EM-wave transmissions and reflections and simultaneously cross-linearized polarization conversions[134]. (c) Design of a multifunctional reconfigurable metasurface for polarization and propagation manipulation[135]. (d) Electrically tunable metasurface absorber based on dissipating behavior of embedded varactors[72]. (e) Active impedance metasurface with full 360° reflection phase tuning[73].](/Images/icon/loading.gif)
Fig. 3. Reconfigurable metasurfaces with active lumped elements. (a) Design of switchable microwave absorber[133]. (b) Single active metasurface to achieve reconfigurable EM-wave transmissions and reflections and simultaneously cross-linearized polarization conversions[134]. (c) Design of a multifunctional reconfigurable metasurface for polarization and propagation manipulation[135]. (d) Electrically tunable metasurface absorber based on dissipating behavior of embedded varactors[72]. (e) Active impedance metasurface with full 360° reflection phase tuning[73].
![Programmable metasurfaces. (a) EM programmable coding metasurface holograms[160]. (b) Reconfigurable water-based metasurface integrated with PIN diodes[79]. (c) Electrically steerable reflector in the microwave regime by incorporating varactor diodes into a reflective array in the metal-insulator-metal (MIM) configuration[161]. (d) Reconfigurable active Huygens metalens[162]. (e) Generation of multiple mode microwave vortex beams using active metasurface[163].](/Images/icon/loading.gif)
Fig. 4. Programmable metasurfaces. (a) EM programmable coding metasurface holograms[160]. (b) Reconfigurable water-based metasurface integrated with PIN diodes[79]. (c) Electrically steerable reflector in the microwave regime by incorporating varactor diodes into a reflective array in the metal-insulator-metal (MIM) configuration[161]. (d) Reconfigurable active Huygens metalens[162]. (e) Generation of multiple mode microwave vortex beams using active metasurface[163].
![Time-modulated metasurfaces. (a) Reflective time-domain digital coding metasurface with independent control of the harmonic amplitude and phase[178]. (b) Time-domain digital coding metasurface with a time-delay gradient[182]. (c) Time-varying, non-reciprocal, reconfigurable metasurface with active switching control of the field-localized waveguide[183]. (d) Anisotropic time-domain digital coding metasurface that can achieve both linear and nonlinear polarization syntheses and realize programmable controls in real time[184]. (e) Time-modulated transparent nonlinear active metasurface loaded with varactor diodes to realize spatial EM wave frequency mixing[185].](/Images/icon/loading.gif)
Fig. 5. Time-modulated metasurfaces. (a) Reflective time-domain digital coding metasurface with independent control of the harmonic amplitude and phase[178]. (b) Time-domain digital coding metasurface with a time-delay gradient[182]. (c) Time-varying, non-reciprocal, reconfigurable metasurface with active switching control of the field-localized waveguide[183]. (d) Anisotropic time-domain digital coding metasurface that can achieve both linear and nonlinear polarization syntheses and realize programmable controls in real time[184]. (e) Time-modulated transparent nonlinear active metasurface loaded with varactor diodes to realize spatial EM wave frequency mixing[185].
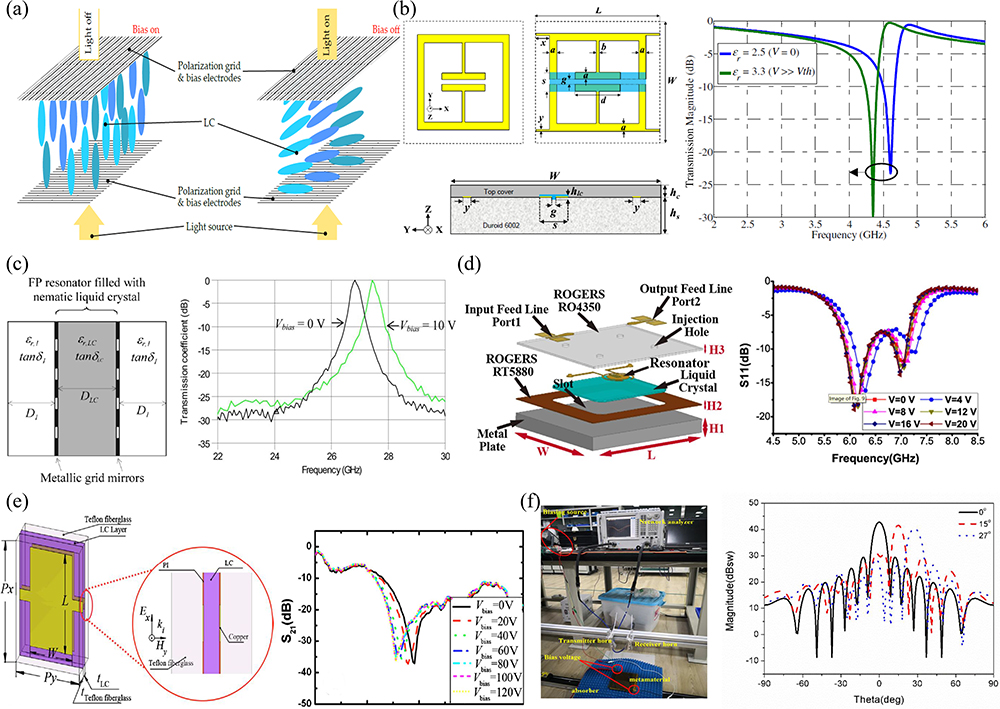
Set citation alerts for the article
Please enter your email address