Baojuan Dong, Teng Yang, Zheng Han. Flattening is flattering: The revolutionizing 2D electronic systems[J]. Chinese Physics B, 2020, 29(9):

Search by keywords or author
- Chinese Physics B
- Vol. 29, Issue 9, (2020)
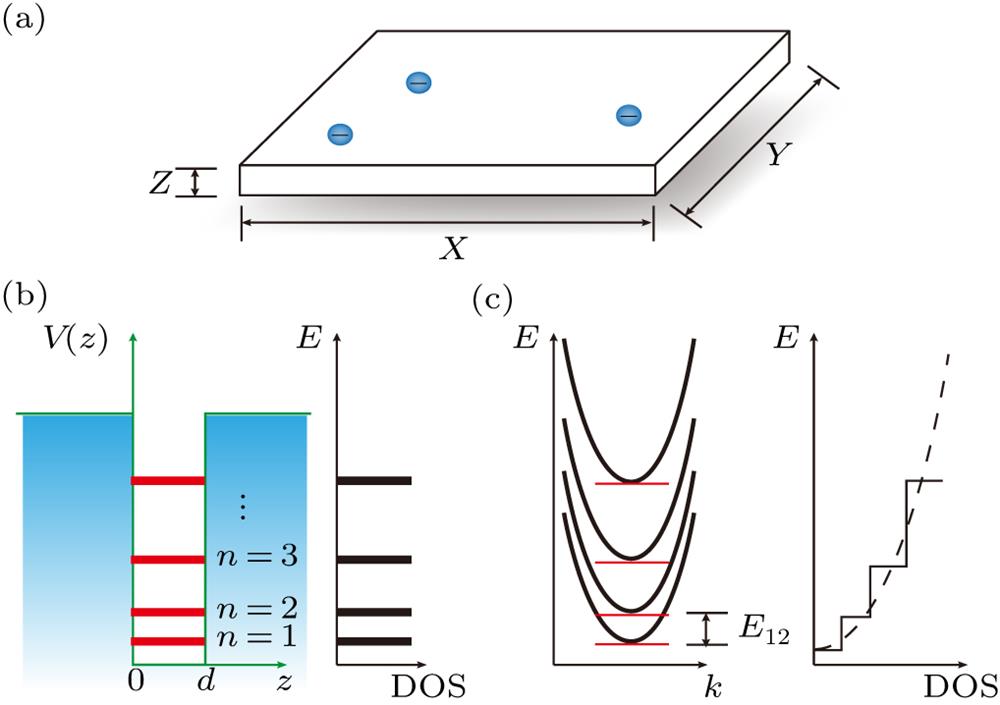
Fig. 1. (a) Schematic 2D structure with z direction confinement. (b) The z -direction confinement spectrum of one-dimensional quantum well model. (c) Band structure and density of states for quasi-two-dimensional electron gas.
![(a) The QHE measured in a 6-nm-thick graphite flake at 0.25 K. (b) Schematic illustrations of electron trajectories under different conditions, (c) Energy gaps for the so-called 2.5D QHE as a function of thickness.[26] Reproduced with permission from Ref. [26].](/richHtml/cpb/2020/29/9/097307/img_2.jpg)
Fig. 2. (a) The QHE measured in a 6-nm-thick graphite flake at 0.25 K. (b) Schematic illustrations of electron trajectories under different conditions, (c) Energy gaps for the so-called 2.5D QHE as a function of thickness.[26 ] Reproduced with permission from Ref. [26 ].
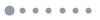
Fig. 3. Schematic illustrations of (a) free 2D electrons, (b) interlayer-interacted 2D electrons, (c) correlated 2D electrons, and (d) twisted 2D electronic systems.
![(a), (b) FeSe monolayer epitaxially grown on SrTiO3(001) substrate, showing superconducting transition temperature above 100 K.[114] (c) Schematic picture of transfer process of isolated freestanding SrTiO3 layers.[115] (d), (e) Characterizations of the SrTiO3 few unit cells.[115] (f)–(h) Superconducting behavior of monolayered BSCCO.[9] Reproduced with permission from Refs. [9,114,115].](/Images/icon/loading.gif)
Fig. 4. (a), (b) FeSe monolayer epitaxially grown on SrTiO3(001) substrate, showing superconducting transition temperature above 100 K.[114 ] (c) Schematic picture of transfer process of isolated freestanding SrTiO3 layers.[115 ] (d), (e) Characterizations of the SrTiO3 few unit cells.[115 ] (f)–(h) Superconducting behavior of monolayered BSCCO.[9 ] Reproduced with permission from Refs. [9 ,114 ,115 ].
![Magnetic hysteresis loops measured in a 5-layered MnBi2Te4, with Hall resistance Rxy quantization indicated by dashed lines in (a) and color map of Rxx in (b).[126] (c)–(e) Morphology of a Sb-doped MnBi2Te4device with 8 monolayers.[127] (f) Phase diagram of Sb-doped MnBi2Te4 in the parameter space of gap energy, doping ratio, and chemical potential.[127] Reproduced with permission from Refs. [126,127].](/Images/icon/loading.gif)
Fig. 5. Magnetic hysteresis loops measured in a 5-layered MnBi2Te4, with Hall resistance Rxy quantization indicated by dashed lines in (a) and color map of Rxx in (b).[126 ] (c)–(e) Morphology of a Sb-doped MnBi2Te4device with 8 monolayers.[127 ] (f) Phase diagram of Sb-doped MnBi2Te4 in the parameter space of gap energy, doping ratio, and chemical potential.[127 ] Reproduced with permission from Refs. [126 ,127 ].
![(a) Schematic picture of a drag system with two separated 2D electrons. (b) Schematic picture of a GaAs/AlGaAs double quantum well sample.[152] (c), (d) Demonstration of Coulomb drag in graphene at zero magnetic field.[147] Width of the Hall bar is about 1.5 μm. (e), (f) Super fluid condensate realized in double bilayer graphene drag system.[142] The excitonic condensate, indicated by the red arrow, was examined in the top drag layer in a counter flow configuration at νT = 1. (b)–(f) Reproduced with permission from Refs. [142,147,152].](/Images/icon/loading.gif)
Fig. 6. (a) Schematic picture of a drag system with two separated 2D electrons. (b) Schematic picture of a GaAs/AlGaAs double quantum well sample.[152 ] (c), (d) Demonstration of Coulomb drag in graphene at zero magnetic field.[147 ] Width of the Hall bar is about 1.5 μm. (e), (f) Super fluid condensate realized in double bilayer graphene drag system.[142 ] The excitonic condensate, indicated by the red arrow, was examined in the top drag layer in a counter flow configuration at ν T = 1. (b)–(f) Reproduced with permission from Refs. [142 ,147 ,152 ].
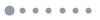
Fig. 7. (a) Schematic picture of a TBLG. (b) Illustration of the electronic band structure of a flat band (blue) induced by the magic angle moiré superlattice. (c) Schematic picture of the moiré superlattice with their stacking order marked with colors. A full filling of the mini flat band corresponds to 4 electrons in the moiré unit cell originated from the 4-fold degeneracy. (d) Summarization of state-of-the-art experimentally observed correlated quantum states in TBLG with the rotation close to the magic angle.
![(a) Schematic picture of a double gate programmable MoS2 transistor, which is capable to integrate photoswitching logic and memory in a single cell.[205] (b) Ballistic avalanche transistor made of InSe/BP heterostructure.[206] (c) Ferroelectric domains realized by local patterning and the resulted photo detector with arbitrarily defined shapes.[207] (d) A FinFET using monolayered TMDC to replace the conventional Si fin channel.[208] Reproduced with permission from Refs. [205–208].](/Images/icon/loading.gif)
Fig. 8. (a) Schematic picture of a double gate programmable MoS2 transistor, which is capable to integrate photoswitching logic and memory in a single cell.[205 ] (b) Ballistic avalanche transistor made of InSe/BP heterostructure.[206 ] (c) Ferroelectric domains realized by local patterning and the resulted photo detector with arbitrarily defined shapes.[207 ] (d) A FinFET using monolayered TMDC to replace the conventional Si fin channel.[208 ] Reproduced with permission from Refs. [205 –208 ].
Set citation alerts for the article
Please enter your email address