Xingyuan Xu, Jiayang Wu, Thach G. Nguyen, Tania Moein, Sai T. Chu, Brent E. Little, Roberto Morandotti, Arnan Mitchell, David J. Moss, "Photonic microwave true time delays for phased array antennas using a 49 GHz FSR integrated optical micro-comb source [Invited]," Photonics Res. 6, B30 (2018)

Search by keywords or author
- Photonics Research
- Vol. 6, Issue 5, B30 (2018)
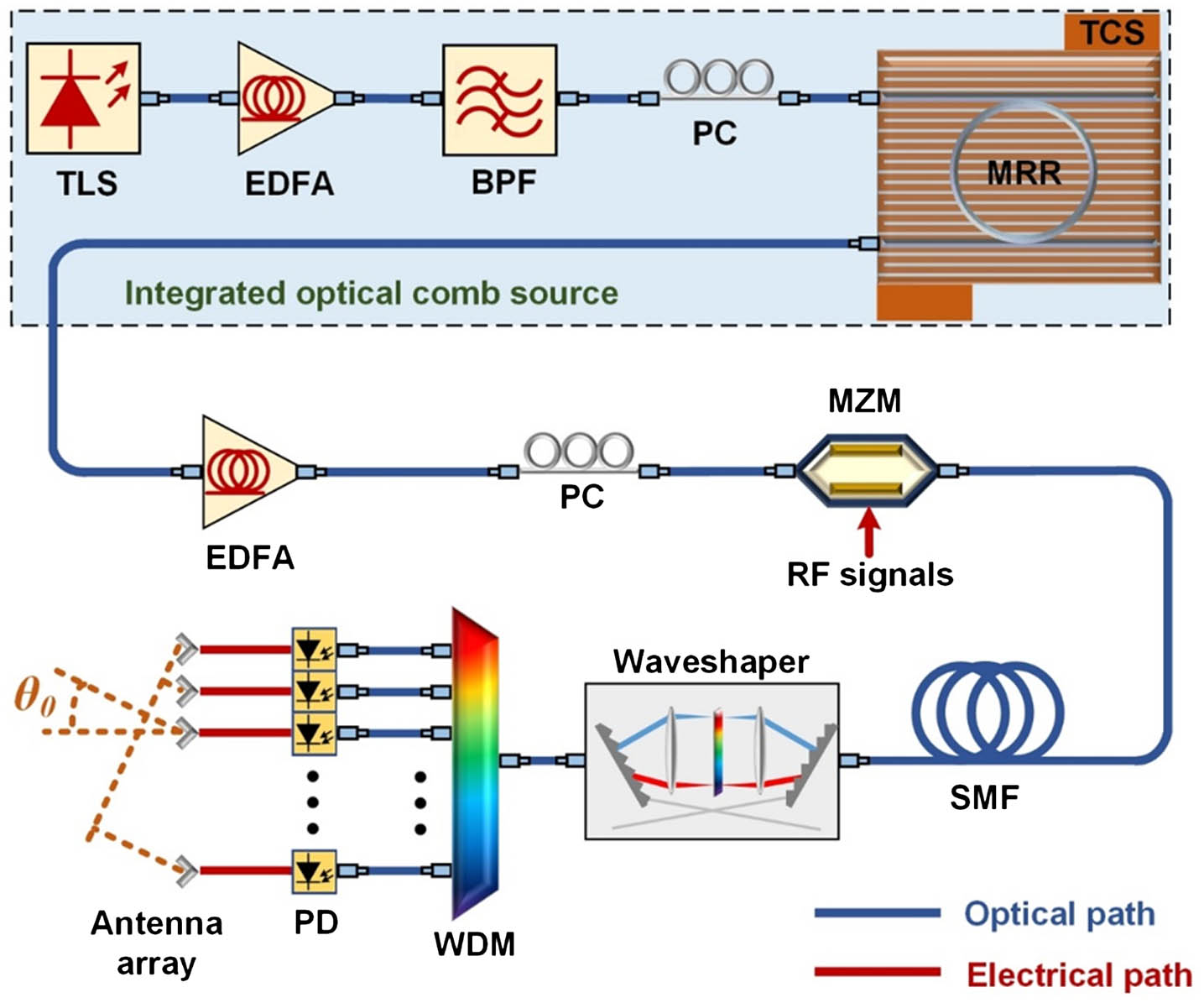
Fig. 1. Scheme of the proposed TTDL based on an integrated optical comb source. TLS, tunable laser source; EDFA, erbium-doped fiber amplifier; BPF, optical bandpass filter; PC, polarization controller; TCS, temperature controller stage; MZM, Mach–Zehnder modulator; SMF, single-mode fiber; WDM, wavelength division multiplexer; PD, photodetector.
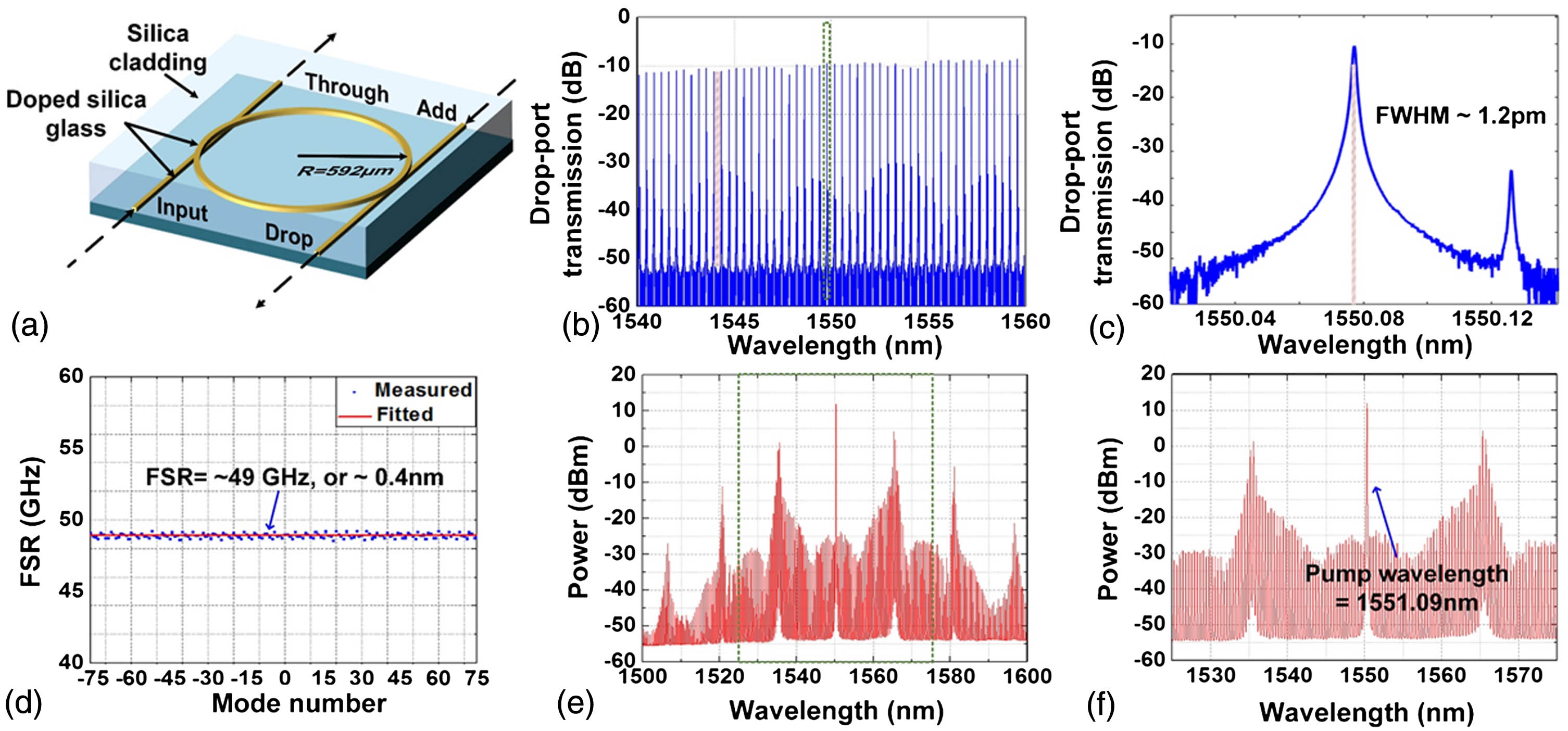
Fig. 2. (a) Schematic illustration of the MRR. Drop-port transmission spectra of the on-chip MRR (b) with a span of 20 nm, showing an FSR of ∼ 0.4 nm , and (c) with a resonance at ∼ 1550 nm with full width at half-maximum (FWHM) of ∼ 1.2 pm (∼ 150 MHz ). (d) Measured and fitted FSR of the MRR. Optical spectra of the generated Kerr comb with a span of (e) 100 nm and (f) 50 nm.
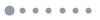
Fig. 3. (a) Measured RF phase response of the 81-channel TTDL and (b) corresponding time delays of each channel. The inset shows flat delays over a wide RF range together with the extracted delay errors. (c) Calculated array factors both with and without delay errors. (d) Calculated array factors with generated weights and with uniform weights. (e) Calculated array factors with M varying from 4 to 81. (f) Relationship between the number of radiating elements (M ) and the 3 dB beamwidth (θ 3 dB ).
![(a) Calculated AFs of the PAA with m varying from 1 to 15 (M=6) based on the 49 GHz FSR Kerr comb. (b) Calculated AFs of the PAA with m varying from 1 to 7 based on a 200 GHz FSR Kerr comb [49]. (c) Calculated AFs of the PAA with m varying from 1 to 27 based on the 49 GHz FSR Kerr comb. (d) Number of radiating elements (M) and the 3 dB beamwidth (θ3 dB) as a function of m. (e) Beam steering angle θ0 as a function of m. (f) Calculated AFs with RF varying from 2 to 17 GHz.](/Images/icon/loading.gif)
Fig. 4. (a) Calculated AFs of the PAA with m varying from 1 to 15 (M = 6 ) based on the 49 GHz FSR Kerr comb. (b) Calculated AFs of the PAA with m varying from 1 to 7 based on a 200 GHz FSR Kerr comb [49]. (c) Calculated AFs of the PAA with m varying from 1 to 27 based on the 49 GHz FSR Kerr comb. (d) Number of radiating elements (M ) and the 3 dB beamwidth (θ 3 dB ) as a function of m . (e) Beam steering angle θ 0 as a function of m . (f) Calculated AFs with RF varying from 2 to 17 GHz.
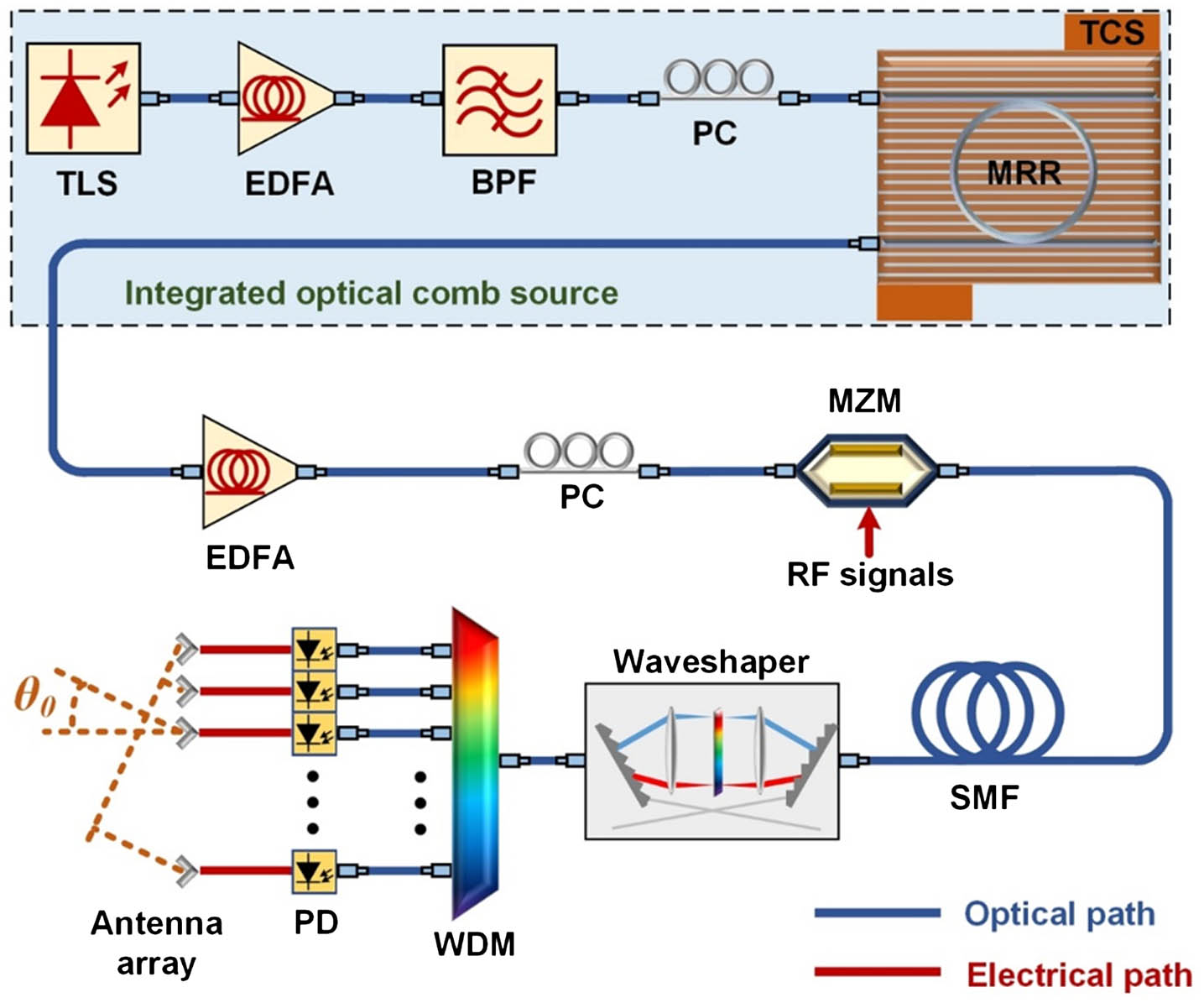
Set citation alerts for the article
Please enter your email address