Wenfeng Cai, Ye Li, Zongyuan Tang, Huilin He, Jiawei Wang, Dan Luo, Yanjun Liu. Liquid Crystal Random Laser: Principles and Research Progresses[J]. Chinese Journal of Lasers, 2021, 48(12): 1201006

Search by keywords or author
- Chinese Journal of Lasers
- Vol. 48, Issue 12, 1201006 (2021)
![Experimental setup for random laser pumping and detection device of DD-NLC [36]](/richHtml/zgjg/2021/48/12/1201006/img_1.jpg)
Fig. 1. Experimental setup for random laser pumping and detection device of DD-NLC [36]
![Emission spectra of 185 μm-thick cell[36]. (a),(b) Emission spectra at different pump energies; (c) emission peak intensity and FWHM of spectra as a function of pumping energies](/richHtml/zgjg/2021/48/12/1201006/img_2.jpg)
Fig. 2. Emission spectra of 185 μm-thick cell[36]. (a),(b) Emission spectra at different pump energies; (c) emission peak intensity and FWHM of spectra as a function of pumping energies
![Random laser spectra under different pump energies[36]](/Images/icon/loading.gif)
Fig. 3. Random laser spectra under different pump energies[36]
![Influences of sample thickness on random laser[36]. (a) Emission spectra with different thicknesses; (b) dependence of emission peak wavelength and average spacing of adjacent narrow spikes on thickness of wedged sample](/Images/icon/loading.gif)
Fig. 4. Influences of sample thickness on random laser[36]. (a) Emission spectra with different thicknesses; (b) dependence of emission peak wavelength and average spacing of adjacent narrow spikes on thickness of wedged sample
![Working principle of PDLC[45]. (a) Off state; (b) on state](/Images/icon/loading.gif)
Fig. 5. Working principle of PDLC[45]. (a) Off state; (b) on state
![Emission spectra of DD-PDLC sample with different pump energies[35]. (a) Pump energy is 20 μJ/pulse; (b) pump energy is 25 μJ/pulse; (c) pump energy is 28 μJ/pulse; (d) pump energy is 35 μJ/pulse; (e) pump energy is 47 μJ/pulse. Insets I and II are emission photo of DD-PDLC sample excited at 40μJ/pulse and a schematic diagram of a closed loop path of light formed by multiple scattering in a DD-PDLC sample, respectively](/Images/icon/loading.gif)
Fig. 6. Emission spectra of DD-PDLC sample with different pump energies[35]. (a) Pump energy is 20 μJ/pulse; (b) pump energy is 25 μJ/pulse; (c) pump energy is 28 μJ/pulse; (d) pump energy is 35 μJ/pulse; (e) pump energy is 47 μJ/pulse. Insets I and II are emission photo of DD-PDLC sample excited at 40μJ/pulse and a schematic diagram of a closed loop path of light formed by multiple scattering in a DD-PDLC sample, respectively
![Output intensity and FWHM of ASE spectra from DD-PDLC sample as functions of laser pump energy[35]](/Images/icon/loading.gif)
Fig. 7. Output intensity and FWHM of ASE spectra from DD-PDLC sample as functions of laser pump energy[35]
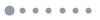
Fig. 8. Schematic diagram of CLC molecular arrangement structure
![Normalized emission spectra from DD-CLC sample when optically excited at λ=532 nm for different applied electric fields[67]. (a) 13 V/μm, 10 Hz; (b) 13 V/μm, 560 Hz; and (c) 13 V/μm, 5 kHz](/Images/icon/loading.gif)
Fig. 9. Normalized emission spectra from DD-CLC sample when optically excited at λ=532 nm for different applied electric fields[67]. (a) 13 V/μm, 10 Hz; (b) 13 V/μm, 560 Hz; and (c) 13 V/μm, 5 kHz
![Optical textures, corresponding diffraction patterns and laser emission spectra of reconstructed CLC under different driven voltages[68]. (a),(d),(g) Applied voltage of 2 V; (b),(e),(h) 3 minutes after applying voltage of 2 V; (c),(f),(i) applied voltage of 10 V followed by a sudden drop to 2 V](/Images/icon/loading.gif)
Fig. 10. Optical textures, corresponding diffraction patterns and laser emission spectra of reconstructed CLC under different driven voltages[68]. (a),(d),(g) Applied voltage of 2 V; (b),(e),(h) 3 minutes after applying voltage of 2 V; (c),(f),(i) applied voltage of 10 V followed by a sudden drop to 2 V
![Images of polarized optical microscope in reflected light mode and emission spectra[79]. (a) Small size pure BPLC platelet (less than 3 μm); (b) intermediate size pure BPLC platelet (10--20 μm); (c) large size pure BPLC platelet (more than 30 μm); (d) intermediate size PS-BPLC platelet (6--15 μm)](/Images/icon/loading.gif)
Fig. 11. Images of polarized optical microscope in reflected light mode and emission spectra[79]. (a) Small size pure BPLC platelet (less than 3 μm); (b) intermediate size pure BPLC platelet (10--20 μm); (c) large size pure BPLC platelet (more than 30 μm); (d) intermediate size PS-BPLC platelet (6--15 μm)
![Laser spectra of PS-BPLC-wedged cell generated from positions of x=0, 2, 4, 6, 8 mm [81]](/Images/icon/loading.gif)
Fig. 12. Laser spectra of PS-BPLC-wedged cell generated from positions of x=0, 2, 4, 6, 8 mm [81]
![Laser spectra of PS-BPLC-wedged cell at position of x=1 mm under different driven voltages[81]](/Images/icon/loading.gif)
Fig. 13. Laser spectra of PS-BPLC-wedged cell at position of x=1 mm under different driven voltages[81]
![Plots of laser emission intensity as a function of pump energy[82]. (a) Laser threshold comparsion between PM597-doped BPI in 100 μm droplet and PM597-doped BPI in 100 μm cell; (b) emission spectra of PM597-doped BPI in 100 μm cell; (c) emission spectra of polyurethane encapsulated BPI droplets of 100 μm in diameter; (d) emission spectra collected across plane of optical pump confirm omnidirectionality of BP droplet](/Images/icon/loading.gif)
Fig. 14. Plots of laser emission intensity as a function of pump energy[82]. (a) Laser threshold comparsion between PM597-doped BPI in 100 μm droplet and PM597-doped BPI in 100 μm cell; (b) emission spectra of PM597-doped BPI in 100 μm cell; (c) emission spectra of polyurethane encapsulated BPI droplets of 100 μm in diameter; (d) emission spectra collected across plane of optical pump confirm omnidirectionality of BP droplet
![Emission spectra of NPDDNLC sample under different conditions[93]. (a) Peak intensity and FWHM as functions of pump energy in NPDDNLC sample; (b) peak intensity and FWHM as functions of pump energy in indye-and-Au-nanoparticle-co-doped ethanol samples; (c) emission spectra of NPDDNLC samples with the cell thickness of 10, 17, 26 μm at fixed pump energy of 8.39 μJ/pulse; (d) output intensity as a function of pump energy in NPDDNLC sample with the cell thickness of 10, 17, 26 μm; (e) spectra of NPDDNLC sample with the cell thickness of 10 μm at fixed pump energy of 17.87 μJ/pulse under different applied electric fields when polarization of the pump light is parallel to rubbing direction; (f) spectra of NPDDNLC sample with the cell thickness of 10 μm at fixed pump energy of 17.87 μJ/pulse under different applied electric fields when polarization of the pump light is perpendicular to rubbing direction. Insets show the output intensity as a function of the applied electric field from 0 V/μm to 2.1 V/μm in (e) and (f)](/Images/icon/loading.gif)
Fig. 15. Emission spectra of NPDDNLC sample under different conditions[93]. (a) Peak intensity and FWHM as functions of pump energy in NPDDNLC sample; (b) peak intensity and FWHM as functions of pump energy in indye-and-Au-nanoparticle-co-doped ethanol samples; (c) emission spectra of NPDDNLC samples with the cell thickness of 10, 17, 26 μm at fixed pump energy of 8.39 μJ/pulse; (d) output intensity as a function of pump energy in NPDDNLC sample with the cell thickness of 10, 17, 26 μm; (e) spectra of NPDDNLC sample with the cell thickness of 10 μm at fixed pump energy of 17.87 μJ/pulse under different applied electric fields when polarization of the pump light is parallel to rubbing direction; (f) spectra of NPDDNLC sample with the cell thickness of 10 μm at fixed pump energy of 17.87 μJ/pulse under different applied electric fields when polarization of the pump light is perpendicular to rubbing direction. Insets show the output intensity as a function of the applied electric field from 0 V/μm to 2.1 V/μm in (e) and (f)
![Schematic diagram of DD-CLC random laser structure and experimental setup[85]. (a) Schematic diagram of DD-CLC random laser structure, in which AgNPs is introduced on P-II glass substrate in liquid crystal cell; (b) generation and measurement devices of DD-CLC random laser, inset shows generated DD-CLC laser](/Images/icon/loading.gif)
Fig. 16. Schematic diagram of DD-CLC random laser structure and experimental setup[85]. (a) Schematic diagram of DD-CLC random laser structure, in which AgNPs is introduced on P-II glass substrate in liquid crystal cell; (b) generation and measurement devices of DD-CLC random laser, inset shows generated DD-CLC laser
![Performance of DD-CLC random laser with different sizes of AgNPs and temperatures[85]. (a) Effects of different sizes of AgNPs on slope efficiency and laser threshold of DD-CLC random laser; (b) temperature-dependent band-edge laser peak positions from S-IV sample; (c) temperature-dependent wavelength shifts of long band-edge laser and short band-edge laser peaks](/Images/icon/loading.gif)
Fig. 17. Performance of DD-CLC random laser with different sizes of AgNPs and temperatures[85]. (a) Effects of different sizes of AgNPs on slope efficiency and laser threshold of DD-CLC random laser; (b) temperature-dependent band-edge laser peak positions from S-IV sample; (c) temperature-dependent wavelength shifts of long band-edge laser and short band-edge laser peaks
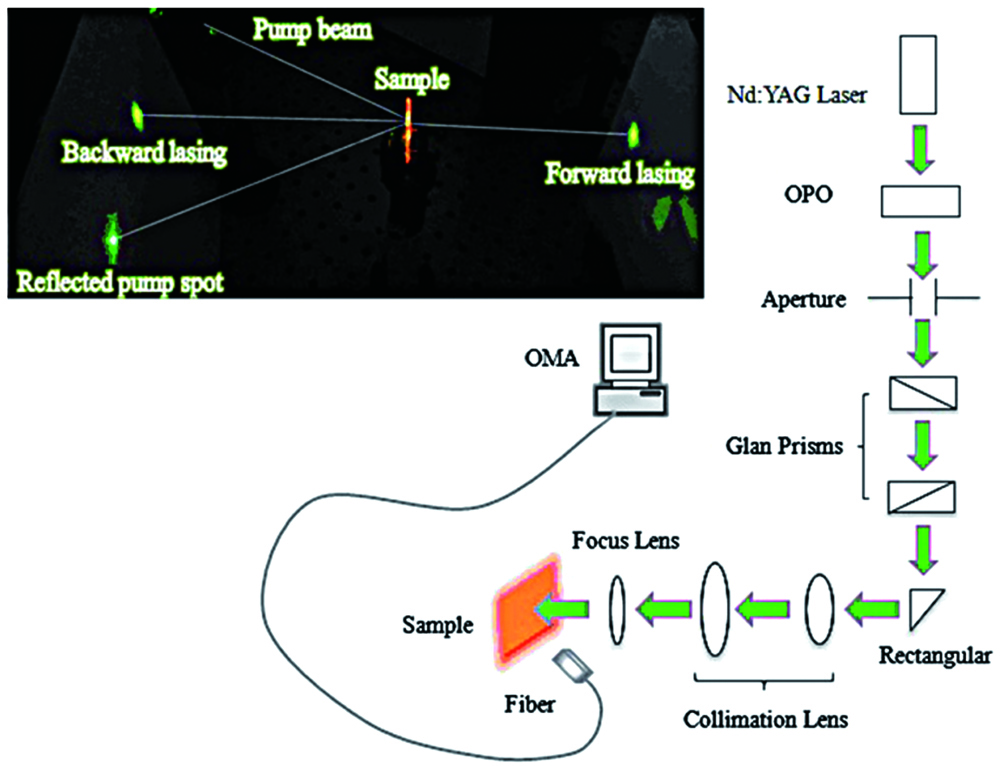
Set citation alerts for the article
Please enter your email address