
- Journal of Inorganic Materials
- Vol. 36, Issue 6, 665 (2021)
Abstract
Keywords
The industrial production processes such as metal smelting, chemical industries, production of pigment, medicine and paper have given rise to heavy metal pollution which are harmful to human beings and other creatures in nature. Among the toxic heavy metal pollutants, the threaten to human being and ecological systems caused by hexavalent chromium (Cr(VI)) has attracted more and more attention since Cr(VI) has high solubility in aqueous solution, substantiated toxicity and potential carcinogenicity. Therefore, it is highly necessary to solve the Cr(VI) presence in wastewater[
Among various photocatalysts, Bi-compounds based photocatalysts, such as Bi2O3, BiVO4, Bi2MoO6, BiOX (X=Cl, Br, I) and Bi2O2CO3 have been extensively reported with satisfactory photocatalytic performance for the degradation of organic pollutants and removal of Cr(VI) reduction[
In addition, CuS, as a p-type photocatalyst with narrow band gap exhibits broad absorption spectra in the range from ultraviolet (UV) to near-infrared (NIR) region, has been extensively used as an efficient photocatalyst. For example, Lai, et al. [
In this work, novel CuS/Bi5O7I composite was fabricated by a facile hydrothermal method. Bi5O7I microrods were decorated by CuS nanosheets, which was in favor of convenient and effective regulation of Bi5O7I-based composite’s photocatalytic properties. The Cr(VI) aqueous solution is employed as the target degradation contamination to evaluate the photocatalytic performance of CuS/Bi5O7I. The photocatalytic experiments results manifested the improved photocatalytic reduction efficiency of CuS/Bi5O7I composites for aqueous Cr(VI) under visible light irradiation. Moreover, the possible mechanism of photocatalytic reduction of Cr(VI) by CuS/Bi5O7I composites was also explored.
1 Experimental
1.1 Synthesis of Bi5O7I microrods
Briefly, Bi(NO3)·5H2O (4.85 g) was dissolved in deionized water (200 mL), ultrasonically dispersed for 30 min and kept stirring for 60 min and then 5 mL of acetic acid was added to obtain suspension A.
KI (1.66 g) was dissolved in deionized water (10 mL) to obtain homogeneous solution which was added to the suspension A dropwise and kept magnetic stirring for 30 min to obtain a black suspension B. After that, 4 mL ammonia water was added dropwise to suspension C until the brick red precipitation appeared. After magnetic stirring at 85 ℃ for 60 min, the BiOI red precipitate was obtained by centrifugation, and fully dried at 70 ℃. The BiOI red powder was then ultrasonically dispersed in a mixture solvent of ethanol and deionized water (25 mL/25 mL) for 30 min to obtain a suspension. Next, NaOH solution (25 mL 0.2 mmol/L) was added to the above suspension with vigorous stirring at 70 ℃ for 60 min. Finally, the white Bi5O7I sample was washed by deionized water and ethanol for several times and dried at 70 ℃ overnight.
1.2 Synthesis of CuS/Bi5O7I
CuS decorated Bi5O7I with different amounts of CuS was prepared as following: CuSO4·5H2O, thiourea and trisodium citrate dihydrate was dissolved in deionized water (30 mL). After stirring for 20 min, Bi5O7I powder were ultrasonically dispersed in the above solution for 30 min. The suspension was then transferred into a 50 mL Teflon-lined stainless autoclave and reacted at 160 ℃ for 10 h. Finally, the CuS/Bi5O7I composite was collected and washed by deionized water, and then dried at 60 ℃ for 10 h. CuS/Bi5O7I composites were denoted as CB-x, where x is the mass percentage (wt%) of CuS, and named as CB-10, CB-15 and CB-20, respectively. The fabrication process of CuS/Bi5O7I composites was presented in Scheme 1. By contrast, pure CuS were also prepared by the procedure described above without the addition of Bi5O7I.
Figure Scheme 1.Preparation process diagram of CuS/Bi5O7I composites
1.3 Characterizations
The phase structure of the samples was determined by the Bruker D8 Advance powder X-ray diffractometer with Cu-Kα irradiation (λ=0.154056 nm). The morphologies and microstructural properties were obtained by field emission scanning electron microscope (FESEM, JSM-7100F, JEOL) and high-resolution transmission electron microscope (HRTEM, JEM 2010F). UV-Vis diffuse reflectance spectra were carried out on a UV-Vis spectrophotometer (UV-3600, Shimadzu) with an integrating sphere attachment. QDS- MP-30 volumetric gas adsorption instrument (Quantachrome, USA) was employed to measure the Brunauer-Emmett- Teller (BET) surface area. X-ray photoelectron spectroscopy (XPS) on an ESCALAB 250 xi apparatus was conducted to reveal the elemental composition and surface states of CuS/Bi5O7I composite. The photoluminescence (PL) spectra were determined by a fluorescence spectrophotometer (PerkinElmer, LS-55) at room temperature. A Zahner CIMPS electrochemical workstation (Germany) was employed to perform the electrochemical impedance spectra (EIS) measurement in a three-electrode system with Pt wire and Ag/AgCl electrode as the counter and reference electrodes, respectively. Pure Bi5O7I, CuS or CB-15 composites spin-coated ITO with the size of 1 cm×1 cm was used as working electrode. The measurement was carried out in 0.2 mol/L Na2SO4 aqueous solution.
1.4 Photocatalytic activity
The photocatalytic properties of the as-synthesized photocatalysts were accessed for the reduction of Cr(VI) to Cr(III) and performed in aqueous solution with concentration of 10 mg/L. The pH of the mixture solution should be controlled at about 3 with H2SO4 solutions. In the photocatalytic activity evaluation experiment, 100 mg sample was added to 150 mL K2Cr2O7 aqueous solutions and kept magnetic stirring for 30 min to reach adsorption/desorption equilibrium. Then, the illumination from a 300 W Xe lamp equipped with 420 nm cutoff filter to make sure the photocatalytic reduction reaction take place under the visible-light irradiation. During the irradiation, 3 mL suspensions were sucked out at given time intervals. After removing the solid photocatalyst by centrifuging and filtration, the concentration of Cr(VI) in the filtrate was measured according to the diphenylcarbazide colorimetric method determined by Shimadzu UV-3600 spectrophotometer. The photocatalytic reduction activity was evaluated by the time-depending C/C0, where C is the remained Cr(VI) concentration and C0 is the initial Cr(VI) concentration.
2 Results and discussion
X-ray diffraction patterns of as-prepared Bi5O7I, CuS and CuS/Bi5O7I composites are presented in Fig. 1. It can be seen that XRD peaks at 2θ=27.9°, 28.9°, 30.9°, 33°, 45.9°, 53.6° and 56.1° correspond to (312), (113), (004), (204), (604), (224), (316) and (912) crystal planes of orthorhombic Bi5O7I, respectively. The synthesized Bi5O7I is well-crystallized, and all of the peaks are indexed to the standard card (JCPDS 40-0548) without other impurity phase[
Figure 1.XRD patterns of Bi5O7I, CuS, and CuS/Bi5O7I composites
SEM images of the pure Bi5O7I and CuS/Bi5O7I composites are presented in Fig. 2. It shows that the synthesized Bi5O7I exhibits a microrod-like morphology with length of about 3-4 μm and diameters of 200-300 nm. The surface of Bi5O7I microrods are smooth which can provide a favorable environment for assembling the co-photocatalyst. After introducing CuS, Bi5O7I microrods are covered by thin CuS nanosheets. The CuS nanosheets grow discretely along the axis of Bi5O7I microrods. In addition, the nanosheets decorated on Bi5O7I microrods become denser with the increasing of CuS mass percentage. Furthermore, the TEM image of CB-15 composite in Fig. 3(a) confirms the SEM results, which illustrates that the surface of Bi5O7I microrods is fully and densely covered by irregular shaped CuS nanosheets with strong linkages in the composite. By analyzing the HRTEM images in Fig. 3(b), the lattice spacing of 0.319 nm corresponds to the (312) plane of orthorhombic Bi5O7I, and the well crystalline lattice fringe with interplanar spacing of 0.304 nm can be ascribed to (102) plane of CuS, respectively. The interface between the Bi5O7I and CuS lattices clearly indicates the formation of CuS/Bi5O7I heterojunctions, which is beneficial for the effective separation of photogenerated electron-hole pairs, and thus enhances the photocatalytic activity[
Figure 2.SEM images of (a) pure Bi5O7I, (b) CB-10, (c) CB-15 and (d) CB-20 composites
Figure 3.(a) TEM and (b) HRTEM images of CB-15 composite
Chemical states and elemental compositions of CB-15 composite were estimated by XPS spectra. It can be observed in Fig. 4(a), the general survey spectrum reveals that the as-prepared CB-15 composite consists of Bi, I, O, Cu and S elements. The signals of C1s located at about 285.3 eV was used for calibration. Fig. 4(b) shows the Bi4f spectrum of CB-15 composite with two peaks at 164.3 and 159.06 eV, which correspond to Bi4f5/2 and Bi4f7/2, respectively, demonstrating the characteristics of Bi3+ ions in the Bi5O7I[
Figure 4.(a) XPS survey spectrum and high resolution XPS spectra of (b) Bi4f, (c) I3d, (d) O1s, (e) Cu2p, and (f) S2s of CB-15 composite
The optical absorption properties of the prepared samples were investigated by UV-Vis diffuse reflectance spectrometer and presented in Fig. 5. Fig. 5(a) indicated that pure Bi5O7I microrods has the absorption edge of about 400 nm with a band gap of 2.92 eV calculated based on Kubelka-Munk theory (Fig. 5(b))[
Figure 5.(a) UV-Vis diffuse reflectance spectra of Bi5O7I, CuS and CuS/Bi5O7I composites, (b) plots of (
Nitrogen adsorption-desorption isotherms analysis was conducted to investigate the specific surface areas of Bi5O7I and CB-15 sample. As shown in Fig. 5(c), the curves of the samples displayed a typical IV feature with a hysteresis loop extended from p/p0 = 0.5 to p/p0 = 1 which indicated mesoporous structures of the samples. The BET surface area of CB-15 is 8.77 m2/g, which is 2.46 times of that of pure Bi5O7I (3.56 m2/g) because the CuS nanosheet decorated Bi5O7I microrods have more stretched surfaces compared with Bi5O7I microrods. The higher surface area of CB-15 composite is usually closely related to more adsorption sites exposed under irradiation and increased adsorption capability which is, therefore, in favor of the enhanced photocatalytic activity.
Photoluminescence (PL) spectra and electrochemical impedance spectroscopy were further employed to analyze the separation efficiency and recombination rate of photoexcited electrons and holes of Bi5O7I and CuS/Bi5O7I composites. It can be observed from Fig. 6(a) that the PL spectra of the as-prepared photocatalysts exhibit an obvious emission peak at about 420 nm. The CuS/Bi5O7I composites exhibit obviously lower intensity than pure Bi5O7I, suggesting that the decoration of CuS nanosheets on Bi5O7I microrods can depress the recombination of photogenerated electrons and holes effectively [
Figure 6.(a) PL spectra and (b) electrochemical impedance spectra of Bi5O7I and CB-15 composite
Electrochemical impedance spectroscopy (EIS) was conducted to testify the charge migration and recommendation process of the photo-induced carriers in CuS/Bi5O7I and at the interface of the composite. The EIS Nyquist plots of Bi5O7I and CB-15 composite as illustrated in Fig. 6(b) show that CB-15 composite possesses smaller arc radius. Generally, the smaller radius implies a lower charge transfer resistance[
On the basis of the analysis from PL spectra and EIS, the improved photocatalytic reduction of Cr(VI) by the CuS/Bi5O7I composite can be attributed to the synergism of the greater surface areas, broader light adsorption and higher separation and transfer efficiency of charge carrier with the CuS decoration on Bi5O7I microrods.
Cr(VI) aqueous solutions were used to assess the photocatalytic activity of the CuS/Bi5O7I composites, as well as pure Bi5O7I and pure CuS. The experiments were carried out under visible light illumination and the results are shown in Fig. 7(a). As can be observed that pure Bi5O7I shows low photocatalytic activity with only 12% efficiency after 4 h of visible-light irradiation which can be attributed to the weak absorption in the visible light range. The photoreduction efficiency of Cr(VI) is about 45% in the presence of pure CuS. Under the identical condition, the removal efficiency of Cr(VI) by CuS/Bi5O7I was noticeably enhanced, which could be attributed to the higher separation efficiency of photo-excited electrons and holes for the CuS/Bi5O7I. On the other hand, it can be obviously found that the photocatalytic activities of CuS/Bi5O7I are dependent on CuS content. With the CuS content increasing from 10wt% to 15wt%, the photocatalytic activity reached the maximum with efficiency of about 92%. However, photocatalytic activity decreased with the CuS content further increasing to 20wt%, which indicated that the proper CuS proportion was conducive to the transfer of electrons and holes at interfaces[
Figure 7.(a) Photocatalytic efficiency, (b) corresponding kinetic curves for the photocatalytic reduction of aqueous Cr(VI) with Bi5O7I and CuS/Bi5O7I composites (experimental conditions: 150 mL of 10 mg/L Cr(IV) solution, 100 mg of catalyst)
Based on the experimental results and discussion above, the photo-induced electron-hole pairs separation process during the reduction of Cr(VI) by CuS/Bi5O7I composite under visible light irradiation was shown in Fig. 8. In general, upon visible illumination, the electrons of Bi5O7I and CuS on the valence band (VB) are excited to conduction band (CB), generating holes on the VB which form large number of valence band.
Figure 8.Schematic diagram of proposed mechanism for photocatalytic reduction of Cr(VI) for CuS/Bi5O7I composite under visible light illumination
The CB edge potential of Bi5O7I is more negative than that of CuS, and the VB position of CuS is positive than that of Bi5O7I. As a result, the photoinduced electrons on the Bi5O7I nanosheet surface can jump easily to the conduction band of CuS resulting in the reduction of Cr2O72- to Cr3+. By this means, the photo-excited electrons and holes are separated effectively in the CuS/Bi5O7I composites and electron-hole recombination behavior is depressed, leading to higher photocatalytic reduction efficiency of Cr(VI) to Cr(III). Consequently, the as-fabricated CuS/Bi5O7I composite photocatalyst shows enhanced photocatalytic performance than Bi5O7I and CuS.
3 Conclusions
In conclusion, CuS nanosheets decorated Bi5O7I composites have been successfully synthesized via a facile solution method. CuS/Bi5O7I composites exhibit a satisfactory strengthened photocatalytic efficiency for the removal of aqueous Cr(VI) comparing with pure Bi5O7I and CuS under visible-light illumination. The photocatalytic reduction rate of Cr(VI) in the presence of CuS/Bi5O7I composite with 15wt% CuS is about 20 times of pristine Bi5O7I and 3 times of pure CuS. The enhanced photocatalytic activity of CuS/Bi5O7I composites is owing to the synergistic effect of the greater surface areas, broader light absorption region, improved charge separation and transfer efficiency of photo-excited electron-hole pairs. This work is helpful for the construction of visible light responsive photocatalytic systems for the practical application involving the treatment of environmental remediation and water purification.
References
[1] R ZHU K, Y GAO, X TAN et al. Polyaniline modified Mg/Al layered double hydroxide composites and their application in efficient removal of Cr(VI). ACS Sustainable Chemistry & Engineering, 4, 4361-4369(2016).
[12] J WU G, Y ZHAO, W LI Y et al. Assembled and isolated Bi5O7I nanowires with good photocatalytic activities. CrystEngComm, 17, 2113-2125(2017).
[14] J DONG Z, Q PAN J, B WANG B et al. The p-n-type Bi5O7I-modified porous C3N4 nano-heterojunction for enhanced visible light photocatalysis. Journal of Alloys & Compounds, 747, 788-795(2018).
[17] R MA, S ZHANG, L LI et al. Enhanced visible-light-induced photoactivity of Type-II CeO2/g-C3N4 nanosheet towards organic pollutants degradation. ACS Sustainable Chemistry & Engineering, 7, 9699-9708(2019).
[23] X SUN L, H SUN J, N HAN et al. rGO decorated W doped BiVO4 novel material for sensing detection of trimethylamine. Sensors & Actuators B: Chemical, 298, 126749(2019).
[24] Q LI, J DU X, Q XIA C et al. Fabrication and photocatalytic properties of nano CuS/MoS2 composite catalyst by dealloying amorphous Ti-Cu-Mo alloy. Applied Surface Science, 221-228(2019).
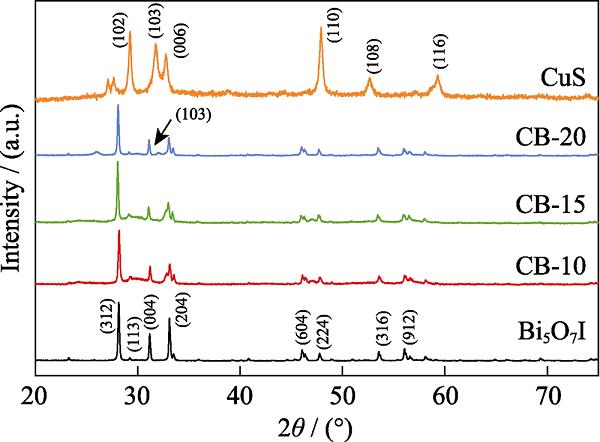
Set citation alerts for the article
Please enter your email address