
- High Power Laser Science and Engineering
- Vol. 6, Issue 4, 04000e55 (2018)
Abstract
1 Introduction
Soon after the generation of the first laser in the 1960s, it was recognized that the energetic laser was one of the most promising and potent options to deliver focused power and energy required for inertial confinement fusion (ICF) in the laboratory[
In this paper, the multifunctional experimental platform of the SG-II series facilities is described in Section
2 Status of the multifunctional platform
The overall layout of the multifunctional platform is shown in Figure
Sign up for High Power Laser Science and Engineering TOC. Get the latest issue of High Power Laser Science and Engineering delivered right to you!Sign up now
Facility | Output capability | Feature |
---|---|---|
SG-II facility | Double-pass, co-axial | |
SG-II 9th laser system | MOPA configuration; higher energy; valuable probe beam | |
SG-II UP facility | Large-aperture four-pass | |
Large energy; high focal power density; high signal to noise ratio; OPCPA | ||
SG-II 5 PW system | 5 PW | Ultra-short higher power; OPCPA amplification new function and technology |
Table 1. Output capability and feature of the present facilities.
The SG-II facility with eight beams can deliver 6 kJ
The SG-II 9th system, a master oscillator power amplifier (MOPA) system, can provide 3 kJ
The SG-II UP facility is composed of two parts; the SG-II UP nanosecond facility and the SG-II UP PW picosecond system. The SG-II UP nanosecond facility with eight beams is designed to provide 24 kJ
The SG-II 5 PW system is designed to provide femtosecond multi-petawatt pulses, employing a three-stage non-collinear optical parametric chirped-pulse amplifier (OPCPA). To date, the previous goals for the two optical parametric amplifiers have been fulfilled, and a chirped pulse with energy of 49.7 J and full-width-at-half-maximum (FWHM) spectrum bandwidth of 85 nm has been achieved. After being compressed, the pulse was greater than 37 J in 21 fs (1.76 PW), and the focal spot was
3 Performance of the SG-II UP facility
The SG-II UP facility is characterized as a typical and new multi-pass amplifier system based on a large-aperture plasma electrode Pockels cell (PEPC) at the NLHPLP. It took nearly 6 years to construct and complete the SG-II UP facility engineering and the demand of the output characteristics for the facility was ultimately satisfied. Many advanced technologies have been adopted to support its construction and some critical optical components have been provided by many suppliers to guarantee the laser performance. Two great developments have been achieved, (1) the transition of the main configuration from a co-axis double-pass of the SG-II facility to the four-pass amplification of the SG-II UP nanosecond system and (2) the realization of a high-energy petawatt laser[
The SG-II UP facility has been in normal operation for physical experiments since 2016. The nanosecond and picosecond systems can be fired independently or flexibly combined. The picosecond laser system can be used to conduct five shots for a physical experiment each day. The SG-II UP facility can support a variety of HED physical experiments, such as fast ignition and fast electron acceleration and proton radiography. More recently, the SG-II UP facility completed the first-phase indirect-drive fast ignition integrated experiment. In this experiment, the observed neutron gain reached a value of
3.1 SG-II UP nanosecond system
3.1.1 Optical layout
A schematic diagram of the overall layout of one beamline of the SG-II UP nanosecond system is shown in Figure
3.1.2 Output ability
The SG-II UP nanosecond system with all eight beamlines can be operated at a
The
The laser power balance is one of the primary criteria representing the overall performance of a laser facility. The laser energy stability is a key factor for the final power imbalance, so the laser energy fluctuation must be carefully controlled during the whole laser propagation and amplification. The energy fluctuation of all eight beams of the SG-II UP nanosecond facility for four consecutive shots is shown in Figure
A flexible pulse shaping stability is important for power balance control and at present different pulse shapes can be generated with high accuracy, mainly, the Haan pulse[
It is known that the precise and stable synchronization between different beams is necessary for positive diagnostics, fast ignition research, and high performance implosion research. The SG-II UP facility utilizes the fiber stacker, high-speed electronics, and analog to digital conversion technology[
3.1.3 Main technical developments
Overall, the SG-II UP nanosecond system has driven the fabrication and processing of large optical components[
(1) The introduction to the overall design of a four-pass amplifier configuration based on a large-aperture PEPC is demonstrated. We have written a set of simulation software called Laser Designer[
For the beam quality, a design incorporating whole system image transfer and aberration elimination was adopted. For example, in the preamplifier system, a zoom spatial filter was used to compensate the optical path difference of the whole system, and the image relaying of eight laser beams was consistent. In the same way, the geometric aberration of the preamplifier system was compensated by the combined lens, and the geometric aberration of the main amplifier system was compensated by the non-spherical lens. Therefore, reasonable elimination of aberration for the whole system was achieved.
To minimize the risk of optical damage the design avoided ghost images of the first to third order in the main amplifier system, and ghost images of the first to fourth order in the final optics system. All types of stray light in the optical path were simultaneously blocked and appropriately absorbed. In order to obtain high contrast and square wave pulses required for physical experiments, the characteristics of the laser pulse temporal waveform shaping were analyzed in detail through the constant correction and improvement of the parameters in the software based on a series of laser shots. The simulation results agreed well with the experimental results, as shown in Figure
(2) Flexible control techniques in the front-end, preamplifier system, and a high stability regenerative amplifier were developed. We independently researched and developed a polarization auto-feedback adjustment module based on fiber optic squeezing[
The spatial shaping in the preamplifier system is important for a good near-field distribution of the final output and improved operating fluence. We adopted spatial beam shaping step by step. A binary mask was used in the front of the preamplifier section to form the soft-edge and beam profile from round to square shape, and another binary mask was used at the end of the preamplifier section to further sharpen the intensity soft edge for improving the energy extraction of main amplifier. An optically addressed spatial light modulator (SLM) developed in 2012[
(3) An alignment system has been designed and applied that takes advantage of the imaging characteristics of a multi-pass amplifier. The alignment system[
The alignment system for the main amplifier and harmonic conversion crystals of FOA is defined here. The main amplifier of the SG-II UP nanosecond laser facility is shown in Figure
The CSF alignment package was utilized to align the far field of the four-pass laser beams, as shown in Figure
The TSF alignment package, shown in Figure
(4) Computational optical imaging has been developed for on-shot, near-field, and far-field parameter characterization of high-power lasers[
Figure
(5) The compact FOA was designed and constructed using a wedged focal lens and specific stray light management[
In order to improve the damage threshold of the KDP crystal, the surface qualities of the KDP crystal must be strictly controlled, such as the transmission wavefront, waviness, and roughness. We processed a KDP crystal with ultra-precision single point diamond tools (SPDT). The surface quality results for processing a type II KDP crystal (350 mm
3.2 SG-II UP PW picosecond system
The SG-II UP PW picosecond system uses a hybrid technology combining OPCPA and Nd:glass CPA to reduce the pre-pulses and gain narrowing effects. A schematic diagram of the system is shown in Figure
The first phase of SG-II UP picosecond laser system development was completed in July 2011 and the system realized laser pulse compression energies of
In the process of the construction of the SG-II UP picosecond laser system, many important technical developments and engineering implementations were achieved. Various problems, such as the high-energy acquisition of pulses with wide spectra, the controlling of signal to noise ratio, and focusing and aligning a pulse with a small focal spot size, were solved. Some of these are discussed below.
(1) A stable OPCPA was developed and two-stage OPCPA amplification was employed to amplify chirped pulses to 100 mJ (gain:
(2) Wide spectrum amplification using Nd:glass has been implemented[
(3) The petawatt picosecond compression chamber was designed and constructed to provide a clean vacuum environment for the compression gratings. This was important to solve the problem of irreversible damage to the gratings caused when hydrocarbon is ionized and evaporates to the surface of the grating. The petawatt picosecond compression chamber is the largest non-oil vacuum container used today in high-energy picosecond lasers in China. To reduce the volume, the compression chamber was designed as a rectangular structure, with a length of 13 m and volume of
(4) Adaptive optics has been adopted in the petawatt system. A primary requirement for a petawatt picosecond laser chain is that 50% of the design energy should be contained within a 25-
(5) A target alignment system was established to satisfy the specifications. The petawatt laser auxiliary alignment system (AAS) is shown in Figure
(6) An apparatus to measure the pulse contrast with a high dynamic range has been developed. The apparatus[
4 Recent development of SG-II 5 PW facility
The SG-II 5 PW facility is under construction and aims to produce
As illustrated in Section
In 2017, the SG-II 5 PW facility became operational and was utilized for high-energy physics experiments. The primary results demonstrated that laser proton accelerations of
We have developed integrated software with a user-friendly interface to implement diagnostics, collimation, and measurement so that the real-time data, including the status of affairs (date, time, serial number, and abnormal operation information of every shot), amplified pulse energy, spectrum, pulse waveform, near field, and far field were automatically collected, processed, and stored. In both the pump and signal laser beams of all of the amplifiers, at the incident and output positions auto-collimation systems including the CCD and detectors were installed, which ensured the high OPCPA conversion efficiency and long optical path transmission. The pulse width after compression was monitored by the home-made full-beam single-shot autocorrelator and accurately controlled by a mini-compressor after OPCPA-I. The mini-compressor not only compensated the residual dispersion between the stretcher and main compressor, but also eliminated the parametric fluorescence from the OPCPA, improving the temporal contrast of the pulse. The spatial filter designs were based on the image relay for beam expansion, high spatial frequency noise filter, and amplified optical parametric fluorescence restriction. For better routine operation, each spatial filter was equipped with a computer-controlled auto-collimation system with actively driven mirrors and an online measurement unit. However, the use of large-aperture refractive spatial filters in a broadband ultra-short pulse laser system could introduce considerable chromatic aberration resulting in degradation of the spatiotemporal quality of the output pulse[
At the beginning of 2018, the latest outline of work has been optimized for the first stage of the OPCPA so that its performance has been greatly improved. The prior two LBO crystals (LBO-I and LBO-II) were replaced by BBO crystals with a parametric bandwidth of
5 Summary
The multifunctional platform including a series of high-power laser facilities at NLHPLP is reviewed. The platform has played an important role in the ICF and HED research fields. The SG-II series facilities available to international users can be operated independently or in combination, with the capability of a wide range of pulse widths. As a newly built facility, the SG-II UP facility, consisting of a single petawatt picosecond system and eight-beam nanosecond system, has achieved the required outputs, and several key technologies have been developed to ensure the performance of the facility. In addition, various physical experiments have been performed with the SG-II 5 PW facility. It is expected that the running platform will continue to provide experimental shots for ICF and HED research. The NLHPLP will continue to enhance the abilities of designing, constructing, and operating high-power facilities, enabling possible larger-scale laser drive developments. Meanwhile, in view of the present large challenges in achieving ignition, we will further improve the openness of our facilities to improve international cooperation.
References
[1] J. Nuckolls, L. Wood, A. Theissen, G. Zimmerman. Nature, 239, 139(1972).
[2] J. L. Emmett, J. Nuckolls, L. Wood. Sci. Amer., 230, 24(1974).
[3] J. F. Holzrichter. Nature, 316, 309(1985).
[5] P. Vivini, M. Nicolaizeau. Proc. SPIE, 9345(2015).
[6]
[7] X. T. He, W. Y. Zhang. Eur. Phys. J. D, 44, 227(2007).
[8] X. M. Deng, W. Y. Yu. IEEE J. Quant. Electron., 17, 1650(1981).
[12] Z. Q. Lin, S. J. Wang, D. Y. Fan, Y. Gu, Z. J. Zheng, J. Q. Zhu, J. Zhu, X. J. Cai, G. L. Huang, Y. P. Dai, Y. Yang. High Power Laser Part. Beams, 14, 403(2002).
[13] Y. Zhao. Proc. SPIE, 3047, 54(1997).
[14] J. Zhu. Chin. J. Nature, 28, 271(2006).
[15] X. T. He. J. Phys. Conf. Ser., 688(2016).
[16] G. Xu, T. Wang, Z. Li, Y. Dai, Z. Lin, Y. Gu, J. Zhu. Rev. Laser Eng. Suppl., 36, 1172(2008).
[17] Y. Gao, Z. Cao, X. Yang, W. Ma, B. Zhu, Z. Lin. Conference on Lasers and Electro-Optics Pacific Rim(2015).
[18] J. Q. Zhu, J. Zhu, X. Li, B. Zhu, W. Ma, D. Liu, C. Liu, X. Lu, W. Fan, Z. Liu, D. Zhao, S. Zhou, Y. Zhang, L. Wang, M. Sun, B. Wang, Z. Jiao, L. Ren, G. Zhang, J. Miao, Z. Lin. Proc. SPIE, 10084(2017).
[19] X. Xie, J. Zhu, Q. Yang, J. Kang, H. Zhu, A. Guo, P. Zhu, Q. Gao. Proc. SPIE, 9513(2015).
[20] http://lssf.cas.cn/dzzRegisterController.do?outerelss&flag=99
[24] H. Shu, S. Fu, X. Huang, J. Wu, H. Zhou, J. Ye. Meas. Sci. Technol., 23(2012).
[25] Y. Gu, F. Zhang, L. Shan, B. Bi, J. Chen, L. Wei, J. Li, Z. Song, Z. Liu, Z. Yang, M. Yu, B. Cui, Y. Zhang, H. Liu, D. Liu, W. Wang, Z. Dai, Y. Yang, L. Yang, F. Zhang, X. Wu, K. Du, W. Zhou, L. Cao, B. Zhang, J. Wu, G. Ren, H. Cai, S. Wu, L. Cao, H. Zhang, C. Zhou, X. He. High Power Laser Part. Beams, 27(2015).
[30] W. Y. Zhang, X. T. He. J. Phys. Conf. Ser., 112(2008).
[31] Y. T. Yuan, L. F. Wang, S. Y. Tu, J. F. Wu, Z. R. Cao, X. Y. Zhan, W. H. Ye, S. Y. Liu, S. E. Jiang, Y. K. Ding, W. Y. Miao. Acta Phys. Sin., 63(2014).
[35] Y. Zhu, Q. Su, G. Chen, L. Dai. Sci. Technol. Rev., 3, 30(2012).
[36] D. Pile. Nat. Photon., 5, 644(2011).
[38] Y. Zhao, J. Yang, J. Zhang, G. Yang, M. Wei, G. Xiong, T. Song, Z. Zhang, L. Bao, B. Deng, Y. Li, X. He, C. Li, Y. Mei, R. Yu, S. Jiang, S. Liu, Y. Ding, B. Zhang. Phys. Rev. Lett., 111(2013).
[39] S. E. Jiang, Y. K. Ding, S. Y. Liu, B. H. Zhang, Z. J. Zheng, J. M. Yang, W. Y. Miao, T. X. Huang, J. Y. Zhang, S. W. Li, L. F. Cao. Wuli, 39, 531(2010).
[42] C. Danson, D. Hillier, N. Hopps, D. Neely. High Power Laser Sci. Eng., 3(2015).
[46] J. Zhang, D. Wu, J. Zheng, K. Zheng, Q. Zhu, X. Zhang. Proc. SPIE, 9294(2014).
[47] J. Shao, K. Yi, M. Zhu. Proc. SPIE, 9983(2016).
[48] A. Guo, H. Zhu, Z. Yang, S. Tang, X. Xie, J. Zhu. Acta Opt. Sin., 33(2013).
[49] W. Jiang, Y. Zhang, C. Rao, N. Ling, C. Guan, M. Li, Z. Yang, G. Shi. Acta Opt. Sin., 31(2011).
[50] D. Zhao, L. Wan, Z. Lin, P. Shao, J. Zhu. High Power Laser Sci. Eng., 3, e7(2015).
[51] X. Ouyang, Y. Cui, J. Zhu, B. Zhu, J. Zhu. Appl. Opt., 55, 7538(2016).
[52] C. Liu, L. Ji, L. Yang, D. Zhao, Y. Zhang, D. Liu, B. Zhu, Z. Lin. Appl. Opt., 55, 2800(2016).
[53] Q. Tang, L. Yang, Y. Guo, X. Ouyang, S. Tang, B. Zhu. Chin. J. Lasers, 40(2013).
[54] P. Zeng, D. Liu, Z. Peng, X. Tang, T. Ji, B. Zhu, D. Liu, J. Zhu. Chin. J. Lasers, 41(2014).
[57] Y. Y. Wang, Y. Q. Gao, H. D. Zhu, X. H. Lu, J. Y. Zhang, Y. J. Guo, H. C. Hui, B. Q. Zhu. Acta Phys. Sin., 62(2013).
[58] W. F. Huang, J. F. Wang, X. H. Lu, X. C. Li. Laser Phys., 23(2013).
[59] Z. Ren, J. Zhu, Z. Liu, X. Yang. High Power Laser Sci. Eng., 6, e1(2018).
[60] B. Wang, J. Zhang, S. Shi, K. You, J. Zhu. High Power Laser Sci. Eng., 4, e9(2016).
[61] J. Zhang, Y. Zhang, W. Ma, J. Zhu, D. A. Liu, L. Yang, J. Zhu, Z. Lin. Acta Opt. Sin., 35(2015).
[62] Y. Li, J. Zhu, Y. Wu, X. Jiao, X. Pang. Proc. SPIE, 9621(2015).
[63] Z. Ren, J. Zhu. Proc. SPIE, 8551(2012).
[64] F. Liu, J. Zhu, J. Xu, Q. Shan, K. Xiao, X. Zhang. Chin. Opt. Lett., 10(2012).
[66] D. T. Casey, J. L. Milovich, V. A. Smalyuk, D. S. Clark, H. F. Robey, A. Pak, A. G. MacPhee, K. L. Baker, C. R. Weber, T. Ma, H.-S. Park, T. Döppner, D. A. Callahan, S. W. Haan, P. K. Patel, J. L. Peterson, D. Hoover, A. Nikroo, C. B. Yeamans, F. E. Merrill, P. L. Volegov, D. N. Fittinghoff, G. P. Grim, M. J. Edwards, O. L. Landen, K. N. Lafortune, B. J. MacGowan, C. C. Widmayer, D. B. Sayre, R. Hatarik, E. J. Bond, S. R. Nagel, L. R. Benedetti, N. Izumi, S. Khan, B. Bachmann, B. K. Spears, C. J. Cerjan, M. Gatu Johnson, J. A. Frenje. Phys. Rev. Lett., 115(2012).
[67] Y. Zhang, G. Li, W. Fan, X. Wang, Y. Jiang, Z. Shi. Laser Optoelectron. Prog., 53(2016).
[69] G. W. Zhang, X. Q. Lu, H. B. Cao, X. H. Yin, F. N. Lu, Z. Zhang, J. H. Li, R. G. Wang, W. X. Ma, J. Zhu. Acta Phys. Sin., 61(2012).
[70] P. Zhang, Y. Jiang, J. Wang, W. Fan, X. Li, J. Zhu. High Power Laser Sci. Eng., 5(2017).
[71] C. Wang, H. Wei, Y. Jiang, J. Wang, Z. Qiao, J. Guo, W. Fan, X. Li. Chin. Opt. Lett., 14(2016).
[72] J. F. Wang, X. C. Li, H. Wei, J. Q. Zhu. Chin. Phys. Lett., 25, 524(2008).
[73] D. Huang, W. Fan, X. Li, Z. Lin. Proc. SPIE, 8556(2012).
[74] D. Z. Liu, J. Q. Zhu, R. F. Xu, D. Y. Fan. Opt. Eng., 43, 2066(2004).
[77] H. Wang, C. Liu, X. He, X. Pan, S. Zhou, R. Wu, J. Zhu. High Power Laser Sci. Eng., 2(2014).
[78] H. Tao, S. P. Veetil, X. Pan, C. Liu, J. Zhu. Chin. Opt. Lett., 14(2016).
[79] D. Zhao, R. Wu, Z. Lin, L. Wang, J. Zhu. Proc. SPIE, 9237(2014).
[80] Y. Guo, S. Tang, X. Jiang, Y. Peng, B. Zhu, Z. Lin. High Power Laser Sci. Eng., 2(2014).
[81] H. Hui, J. Zhang, X. Lu, J. Zhang, X. Chen, X. Jiang, B. Zhu, Z. Lin. Chin. Opt. Lett., 14(2016).
[83] L. Ren, P. Shao, D. Zhao, Y. Zhou, Z. Cai, N. Hua, Z. Jiao, L. Xia, Z. Qiao, R. Wu, L. Ji, D. Liu, L. Ju, W. Pan, Q. Li, Q. Ye, M. Sun, J. Zhu, Z. Lin. High Power Laser Sci. Eng., 6(2018).
[84] D. Zhang, L. Qian, P. Yuan, H. Zhu, S. Wen, C. Xu. Opt. Lett., 33, 1969(2008).
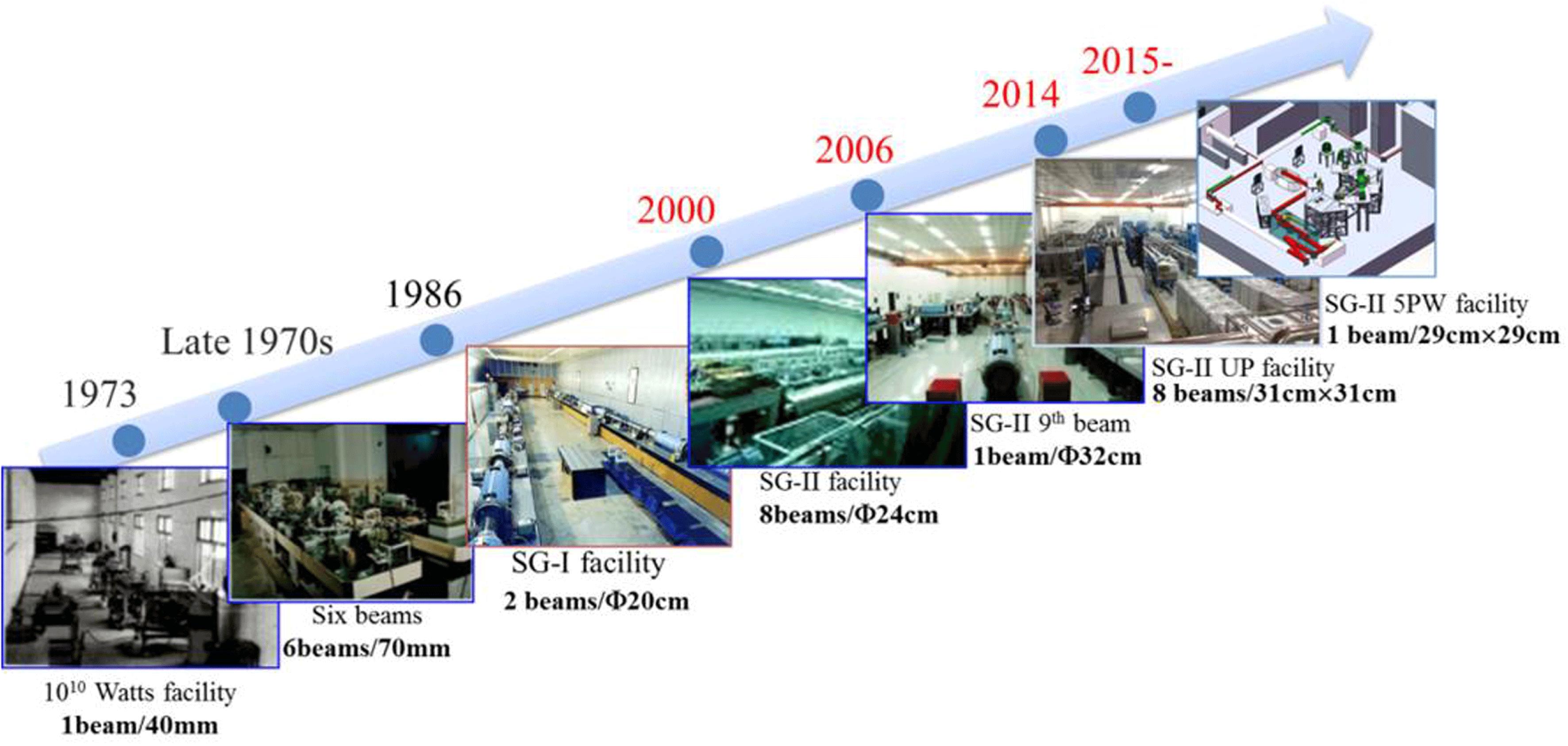
Set citation alerts for the article
Please enter your email address