
- Photonics Research
- Vol. 9, Issue 6, 1048 (2021)
Abstract
1. INTRODUCTION
It is known that angular momentum of light has a spin part associated with polarization [1] and an orbital part associated with spatial distribution [2]. A cylindrical vector beam (CVB) [3–5], acting as a solution of the vectorial Helmholtz equation [6,7], combines the two parts of angular momentum. Radial polarization and azimuthal polarization are the most conspicuous CVBs. Under tight focusing [8–10], radial polarization possesses a sharper focal spot than a homogeneously polarized beam [11,12], while azimuthal polarization can be focused into a hollow spot [13]. These peculiar properties are useful for many applications, such as particle manipulation [14–18], microscopy [19–21], material processing [22–24], near-field optics [25], and nonlinear optics [26]. Recently, the degrees of freedom of CVB are extended by ray-like trajectories [27], so that CVBs also present growing potential in the area of optical encoding [28] and optical communications [29–32].
There are many methods to generate CVBs. Special intracavity resonators could directly generate CVBs from a laser when the cavity geometry is precisely controlled into a frequency-degenerate state [33–37]. Meanwhile, single-element CVB generators have been introduced to tailor Pancharatnam–Berry phases [38–41], supporting modulation of a couple of orthogonal polarization bases with conjugate phase distributions, to generate CVBs from a given basic laser mode. All of these methods need to switch in different elements to generate CVBs with different topological charges, which is inconvenient in experiments that involve multiple CVBs. For example, if there is a circumstance that requests a tunable superposition state of CVBs with arbitrary customized amplitudes on each basis, solid metasurfaces will not be satisfactory. With the same circumstance, implementations containing interferometers, where two orthogonal polarizations are modulated by spatially programmable devices individually and are then combined together [42], still work. Though ingenious devices with high robustness [43–45] are developed, their complexity of modulating two individual parts still sets them as bulky and redundant. Therefore, it is significant to create a compact passive device to generate CVBs with arbitrary topological charges.
In this paper, we provide and demonstrate a passive device to generate CVBs with arbitrary azimuthal and radial topological charges. It is composed with a couple of polarization-selective cylindrical lenses to realize the mode-dependent
Sign up for Photonics Research TOC. Get the latest issue of Photonics Research delivered right to you!Sign up now
2. MAPPING THEORY
Here Laguerre–Gaussian (LG) modes, a complete set for presenting transverse modes, are expressed with complex amplitude:
Figure 1.(a) Basic Poincaré sphere. Selected points a1–a6 on the surface include polarization states from linear polarization to elliptical polarization. (b) First-order HOPS. Selected points b1–b6 represent six CVBs on the surface.
Both radial polarization and azimuthal polarization are located on the equator of first-order HOPS. Denoting
3. IMPLEMENTATION
Significantly, the mapping is characterized by a polarization-selective conversion where
Inspired by the design of the
Figure 2.(a) Fabrication of polarization-selective cylindrical lens. (b) PGPS, which contains two symmetric polarization-selective cylindrical lenses and operates on LCP only. (c) Gouy phases accumulated by PGPS for modes with different indices
The inversion of index
In the following, a polarization-selective device is constructed operating on HG modes (
Figure 3 shows the experimental scheme. He-Ne laser derives a 632.8 nm Gaussian beam whose polarization is projected to horizontal by a half-wave plate (HWP) and a polarizing beam splitter (PBS). Two lenses constitute an expander to provide an almost plane wave for the spatial light modulator (SLM, Holoeye, Pluto-VIS-016). Fork-like holograms [57] are loaded on the screen of the SLM to produce LG modes. The beam splitter (BS) ensures light beam propagates in the correct path. Two lenses and the iris select the first order of diffracted light after the SLM by reducing them into an appropriate scale. The characteristics of the SLM determine that the original polarization of the selected beam is horizontal. If a pre-production of polarization is necessary, a quarter-wave plate (QWP) and an HWP will be included in the optical circuit. Then the
Figure 3.Schematic of the experimental setup for generating arbitrary CVBs.
Figure 4.Stokes parameters of CVBs. The left column displays the tailoring polarization vectors of CVBs, followed by columns of Stokes parameters
As for
Figure 5.Petal-like profiles collected by CCD when launching high-order LG modes. The first row is set for
4. CONCLUSION
We propose and demonstrate a passive device based on
References
[1] R. A. Beth. Mechanical detection and measurement of the angular momentum of light. Phys. Rev., 50, 115-125(1936).
[2] L. Allen, M. W. Beijersbergen, R. Spreeuw, J. Woerdman. Orbital angular momentum of light and the transformation of Laguerre–Gaussian laser modes. Phys. Rev. A, 45, 8185-8189(1992).
[3] I. Freund. Polarization flowers. Opt. Commun., 199, 47-63(2001).
[4] Q. Zhan. Cylindrical vector beams: from mathematical concepts to applications. Adv. Opt. Photon., 1, 1-57(2009).
[5] X.-L. Wang, Y. Li, J. Chen, C.-S. Guo, J. Ding, H.-T. Wang. A new type of vector fields with hybrid states of polarization. Opt. Express, 18, 10786-10795(2010).
[6] D. G. Hall. Vector-beam solutions of Maxwell’s wave equation. Opt. Lett., 21, 9-11(1996).
[7] X.-L. Wang, J. Ding, W.-J. Ni, C.-S. Guo, H.-T. Wang. Generation of arbitrary vector beams with a spatial light modulator and a common path interferometric arrangement. Opt. Lett., 32, 3549-3551(2007).
[8] J. Pu, Z. Zhang. Tight focusing of spirally polarized vortex beams. Opt. Laser Technol., 42, 186-191(2010).
[9] A. P. Porfirev, A. V. Ustinov, S. N. Khonina. Polarization conversion when focusing cylindrically polarized vortex beams. Sci. Rep., 6, 6(2016).
[10] E. Otte, K. Tekce, C. Denz. Tailored intensity landscapes by tight focusing of singular vector beams. Opt. Express, 25, 20194-20201(2017).
[11] K. S. Youngworth, T. G. Brown. Focusing of high numerical aperture cylindrical-vector beams. Opt. Express, 7, 77-87(2000).
[12] R. Dorn, S. Quabis, G. Leuchs. Sharper focus for a radially polarized light beam. Phys. Rev. Lett., 91, 233901(2003).
[13] Q. Zhan, J. R. Leger. Focus shaping using cylindrical vector beams. Opt. Express, 10, 324-331(2002).
[14] D. G. Grier. A revolution in optical manipulation. Nature, 424, 810-816(2003).
[15] Q. Zhan. Trapping metallic Rayleigh particles with radial polarization. Opt. Express, 12, 3377-3382(2004).
[16] Z. Man, Z. Bai, J. Li, S. Zhang, X. Li, Y. Zhang, X. Ge, S. Fu. Optical cage generated by azimuthal-and radial-variant vector beams. Appl. Opt., 57, 3592-3597(2018).
[17] A. Turpin, V. Shvedov, C. Hnatovsky, Y. V. Loiko, J. Mompart, W. Krolikowski. Optical vault: a reconfigurable bottle beam based on conical refraction of light. Opt. Express, 21, 26335-26340(2013).
[18] X. Weng, X. Gao, H. Guo, S. Zhuang. Creation of tunable multiple 3D dark spots with cylindrical vector beam. Appl. Opt., 53, 2470-2476(2014).
[19] S. W. Hell, J. Wichmann. Breaking the diffraction resolution limit by stimulated emission: stimulated-emission-depletion fluorescence microscopy. Opt. Lett., 19, 780-782(1994).
[20] D. P. Biss, K. S. Youngworth, T. G. Brown. Dark-field imaging with cylindrical-vector beams. Appl. Opt., 45, 470-479(2006).
[21] L. Novotny, M. Beversluis, K. Youngworth, T. Brown. Longitudinal field modes probed by single molecules. Phys. Rev. Lett., 86, 5251-5254(2001).
[22] M. Kraus, M. A. Ahmed, A. Michalowski, A. Voss, R. Weber, T. Graf. Microdrilling in steel using ultrashort pulsed laser beams with radial and azimuthal polarization. Opt. Express, 18, 22305-22313(2010).
[23] C. Hnatovsky, V. G. Shvedov, W. Krolikowski. The role of light-induced nanostructures in femtosecond laser micromachining with vector and scalar pulses. Opt. Express, 21, 12651-12656(2013).
[24] J. Wang, F. Castellucci, S. Franke-Arnold. Vectorial light–matter interaction: exploring spatially structured complex light fields. AVS Quantum Sci., 2, 031702(2021).
[25] A. Ciattoni, B. Crosignani, P. Di Porto, A. Yariv. Azimuthally polarized spatial dark solitons: exact solutions of Maxwell’s equations in a Kerr medium. Phys. Rev. Lett., 94, 073902(2005).
[26] A. Bouhelier, M. Beversluis, A. Hartschuh, L. Novotny. Near-field second-harmonic generation induced by local field enhancement. Phys. Rev. Lett., 90, 013903(2003).
[27] Y. Shen, X. Yang, D. Naidoo, X. Fu, A. Forbes. Structured ray-wave vector vortex beams in multiple degrees of freedom from a laser. Optica, 7, 820-831(2020).
[28] G. Milione, T. A. Nguyen, J. Leach, D. A. Nolan, R. R. Alfano. Using the nonseparability of vector beams to encode information for optical communication. Opt. Lett., 40, 4887-4890(2015).
[29] G. Gibson, J. Courtial, M. J. Padgett, M. Vasnetsov, V. Pas’ko, S. M. Barnett, S. Franke-Arnold. Free-space information transfer using light beams carrying orbital angular momentum. Opt. Express, 12, 5448-5456(2004).
[30] N. Bozinovic, Y. Yue, Y. Ren, M. Tur, P. Kristensen, H. Huang, A. E. Willner, S. Ramachandran. Terabit-scale orbital angular momentum mode division multiplexing in fibers. Science, 340, 1545-1548(2013).
[31] G. Milione, M. P. Lavery, H. Huang, Y. Ren, G. Xie, T. A. Nguyen, E. Karimi, L. Marrucci, D. A. Nolan, R. R. Alfano, A. E. Willner. 4×20 gbit/s mode division multiplexing over free space using vector modes and a
[32] J. Jia, Z. Chang, H. Yang, Q. Liu, F. Wang, H. Gao, F. Li, P. Zhang. Mode sorter designed for (de)multiplexing vector vortex modes. Appl. Opt., 58, 7094-7099(2019).
[33] Y. Kozawa, S. Sato. Generation of a radially polarized laser beam by use of a conical Brewster prism. Opt. Lett., 30, 3063-3065(2005).
[34] M. A. Ahmed, A. Voss, M. M. Vogel, T. Graf. Multilayer polarizing grating mirror used for the generation of radial polarization in Yb:YAG thin-disk lasers. Opt. Lett., 32, 3272-3274(2007).
[35] D. Naidoo, F. S. Roux, A. Dudley, I. Litvin, B. Piccirillo, L. Marrucci, A. Forbes. Controlled generation of higher-order Poincaré sphere beams from a laser. Nat. Photonics, 10, 327-332(2016).
[36] D. Wei, Y. Cheng, R. Ni, Y. Zhang, X. Hu, S. Zhu, M. Xiao. Generating controllable Laguerre–Gaussian laser modes through intracavity spin-orbital angular momentum conversion of light. Phys. Rev. Appl., 11, 014038(2019).
[37] Z. Wang, Y. Shen, D. Naidoo, X. Fu, A. Forbes. Astigmatic hybrid SU(2) vector vortex beams: towards versatile structures in longitudinally variant polarized optics. Opt. Express, 29, 315-329(2021).
[38] L. Marrucci, C. Manzo, D. Paparo. Optical spin-to-orbital angular momentum conversion in inhomogeneous anisotropic media. Phys. Rev. Lett., 96, 163905(2006).
[39] F. Cardano, E. Karimi, S. Slussarenko, L. Marrucci, C. de Lisio, E. Santamato. Polarization pattern of vector vortex beams generated by
[40] J. B. Mueller, N. A. Rubin, R. C. Devlin, B. Groever, F. Capasso. Metasurface polarization optics: independent phase control of arbitrary orthogonal states of polarization. Phys. Rev. Lett., 118, 113901(2017).
[41] R. C. Devlin, A. Ambrosio, N. A. Rubin, J. B. Mueller, F. Capasso. Arbitrary spin-to–orbital angular momentum conversion of light. Science, 358, 896-901(2017).
[42] C. Rosales-Guzmán, N. Bhebhe, A. Forbes. Simultaneous generation of multiple vector beams on a single SLM. Opt. Express, 25, 25697-25706(2017).
[43] H. Chen, J. Hao, B.-F. Zhang, J. Xu, J. Ding, H.-T. Wang. Generation of vector beam with space-variant distribution of both polarization and phase. Opt. Lett., 36, 3179-3181(2011).
[44] S. Chen, X. Zhou, Y. Liu, X. Ling, H. Luo, S. Wen. Generation of arbitrary cylindrical vector beams on the higher order Poincaré sphere. Opt. Lett., 39, 5274-5276(2014).
[45] R. Liu, L.-J. Kong, W.-R. Qi, S.-Y. Huang, Z.-X. Wang, C. Tu, Y. Li, H.-T. Wang. Compact, robust, and high-efficiency generator of vector optical fields. Opt. Lett., 44, 2382-2385(2019).
[46] M. W. Beijersbergen, L. Allen, H. Van der Veen, J. Woerdman. Astigmatic laser mode converters and transfer of orbital angular momentum. Opt. Commun., 96, 123-132(1993).
[47] J. Jia, Q. Li, K. Zhang, D. Chen, C. Wang, H. Gao, F. Li, P. Zhang. Integrated design of π/2 converter and its experimental performance. Appl. Opt., 57, 6076-6082(2018).
[48] G. Milione, H. Sztul, D. Nolan, R. Alfano. Higher-order Poincaré sphere, Stokes parameters, and the angular momentum of light. Phys. Rev. Lett., 107, 053601(2011).
[49] A. A. Tovar. Production and propagation of cylindrically polarized Laguerre–Gaussian laser beams. J. Opt. Soc. Am. A, 15, 2705-2711(1998).
[50] R. Simon, H. Kimble, E. Sudarshan. Evolving geometric phase and its dynamical manifestation as a frequency shift: an optical experiment. Phys. Rev. Lett., 61, 19-22(1988).
[51] P. G. Kwiat, R. Y. Chiao. Observation of a nonclassical Berry’s phase for the photon. Phys. Rev. Lett., 66, 588-591(1991).
[52] Z. Bomzon, V. Kleiner, E. Hasman. Pancharatnam–Berry phase in space-variant polarization-state manipulations with subwavelength gratings. Opt. Lett., 26, 1424-1426(2001).
[53] Z. Bomzon, G. Biener, V. Kleiner, E. Hasman. Space-variant Pancharatnam–Berry phase optical elements with computer-generated subwavelength gratings. Opt. Lett., 27, 1141-1143(2002).
[54] C. Oh, M. J. Escuti. Achromatic diffraction from polarization gratings with high efficiency. Opt. Lett., 33, 2287-2289(2008).
[55] E. Hasman, V. Kleiner, G. Biener, A. Niv. Polarization dependent focusing lens by use of quantized Pancharatnam–Berry phase diffractive optics. Appl. Phys. Lett., 82, 328-330(2003).
[56] S. Feng, H. G. Winful. Physical origin of the Gouy phase shift. Opt. Lett., 26, 485-487(2001).
[57] E. Bolduc, N. Bent, E. Santamato, E. Karimi, R. W. Boyd. Exact solution to simultaneous intensity and phase encryption with a single phase-only hologram. Opt. Lett., 38, 3546-3549(2013).
[58] B. Schaefer, E. Collett, R. Smyth, D. Barrett, B. Fraher. Measuring the Stokes polarization parameters. Am. J. Phys., 75, 163-168(2007).
[59] J. Wang, X. Yang, Y. Li, Y. Chen, M. Cao, D. Wei, H. Gao, F. Li. Optically spatial information selection with hybridly polarized beam in atomic vapor. Photon. Res., 6, 451-456(2018).
[60] X. Yi, Y. Liu, X. Ling, X. Zhou, Y. Ke, H. Luo, S. Wen, D. Fan. Hybrid-order Poincaré sphere. Phys. Rev. A, 91, 023801(2015).
[61] Z. Liu, Y. Liu, Y. Ke, Y. Liu, W. Shu, H. Luo, S. Wen. Generation of arbitrary vector vortex beams on hybrid-order Poincaré sphere. Photon. Res., 5, 15-21(2017).
[62] J. Wang, J.-Y. Yang, I. M. Fazal, N. Ahmed, Y. Yan, H. Huang, Y. Ren, Y. Yue, S. Dolinar, M. Tur, A. E. Willner. Terabit free-space data transmission employing orbital angular momentum multiplexing. Nat. Photonics, 6, 488-496(2012).
[63] Y. Zhou, M. Mirhosseini, S. Oliver, J. Zhao, S. M. H. Rafsanjani, M. P. Lavery, A. E. Willner, R. W. Boyd. Using all transverse degrees of freedom in quantum communications based on a generic mode sorter. Opt. Express, 27, 10383-10394(2019).
[64] F. Wang, P. Zeng, J. Zhao, B. Braverman, Y. Zhou, M. Mirhosseini, X. Wang, H. Gao, F. Li, R. W. Boyd, P. Zhang. High-dimensional quantum key distribution based on mutually partially unbiased bases. Phys. Rev. A, 101, 032340(2020).
[65] D. Lin, P. Fan, E. Hasman, M. L. Brongersma. Dielectric gradient metasurface optical elements. Science, 345, 298-302(2014).
[66] G. Hu, X. Hong, K. Wang, J. Wu, H.-X. Xu, W. Zhao, W. Liu, S. Zhang, F. Garcia-Vidal, B. Wang, P. Lu, C. Qiu. Coherent steering of nonlinear chiral valley photons with a synthetic Au-WS2 metasurface. Nat. Photonics, 13, 467-472(2019).
[67] G. Hu, M. Wang, Y. Mazor, C. Qiu, A. Alã. Tailoring light with layered and Moiré metasurfaces. Trends Chem., 3, 342-358(2021).
[68] F. Aieta, P. Genevet, M. A. Kats, N. Yu, R. Blanchard, Z. Gaburro, F. Capasso. Aberration-free ultrathin flat lenses and axicons at telecom wavelengths based on plasmonic metasurfaces. Nano Lett., 12, 4932-4936(2012).
[69] M. Khorasaninejad, W. T. Chen, R. C. Devlin, J. Oh, A. Y. Zhu, F. Capasso. Metalenses at visible wavelengths: diffraction-limited focusing and subwavelength resolution imaging. Science, 352, 1190-1194(2016).
[70] A. Y. Piggott, J. Lu, K. G. Lagoudakis, J. Petykiewicz, T. M. Babinec, J. Vučković. Inverse design and demonstration of a compact and broadband on-chip wavelength demultiplexer. Nat. Photonics, 9, 374-377(2015).
[71] J. Chen, X. Chen, T. Li, S. Zhu. On-chip detection of orbital angular momentum beam by plasmonic nanogratings. Laser Photon. Rev., 12, 1700331(2018).
[72] K. Wei, W. Li, H. Tan, Y. Li, H. Min, W.-J. Zhang, H. Li, L. You, Z. Wang, X. Jiang, T. Chen, S. Liao, C. Peng, F. Xu, J. Pan. High-speed measurement-device-independent quantum key distribution with integrated silicon photonics. Phys. Rev. X, 10, 031030(2020).
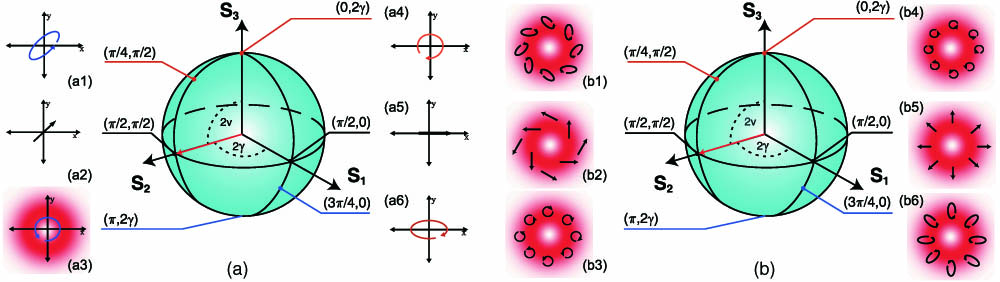
Set citation alerts for the article
Please enter your email address