D. Raffestin, L. Lecherbourg, I. Lantuéjoul, B. Vauzour, P. E. Masson-Laborde, X. Davoine, N. Blanchot, J. L. Dubois, X. Vaisseau, E. d’Humières, L. Gremillet, A. Duval, Ch. Reverdin, B. Rosse, G. Boutoux, J. E. Ducret, Ch. Rousseaux, V. Tikhonchuk, D. Batani. Enhanced ion acceleration using the high-energy petawatt PETAL laser[J]. Matter and Radiation at Extremes, 2021, 6(5): 056901

Search by keywords or author
- Matter and Radiation at Extremes
- Vol. 6, Issue 5, 056901 (2021)
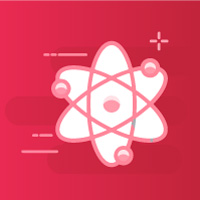
Fig. 1. Focal spot measured after the compression stage in high-energy shot #176 (450 J energy and 610 fs duration).
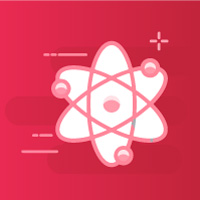
Fig. 2. Tω ist image (a) and horizontal lineout (b) of the on-target focal spot in shot #178 (187 J/610 fs).
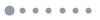
Fig. 3. Experimental setup and positioning of diagnostics within the LMJ chamber (angles are relative to the PETAL laser beam direction).
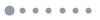
Fig. 4. Electron energy spectra measured at 13.5° and 58.5° from the rear target normal by SESAME on shot #176.
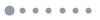
Fig. 5. Electron energy spectra measured at 13.5° by SESAME on shots #176, #177, and #178.
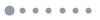
Fig. 6. Thomson parabola traces (high-energy SEPAGE channel) obtained on an MS imaging plate in shot #176: 450 J/610 fs laser pulse on a 50 µ m thick plastic target (CH + 1 µ m Al coating).
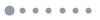
Fig. 7. C4+, C5+, and O6+ spectra (uncalibrated) for shot #176.
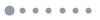
Fig. 8. Comparison of proton spectra obtained on the SEPAGE Thomson parabola and RCF stacks for shot #176, #177, and #178.
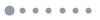
Fig. 9. (a) RCF images (EBT3) due to protons obtained in shot #176: 450 J/610 fs on a 50 µ m thick plastic target (CH + 1 µ m Al). (b) Annular distribution pattern on HDV2 RCF (protons around 9 MeV).
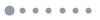
Fig. 10. PETAL prepulse contrast (ratio of intensity to peak intensity): long-term (5 ns) and short-term (in the inset, >250 ps) before the main pulse.
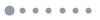
Fig. 11. (a) Lineout of electron density (cm−3) along the laser propagation axis for two different laser energies (the inset shows a zoom of the dashed rectangle), just before the arrival of the main PETAL pulse. The laser is coming from the left side, and the target was initially located between the positions 142 and 192 µ m. (b) Electron density profile assuming full ionization of the plasma: the profile obtained from the TROLL simulation with 450 J (blue) and the fit used to initialize the PIC simulation (red).
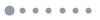
Fig. 12. Average laser amplitude distribution in the preplasma at t = 1 ps. The laser beam propagates from left to right. The preplasma density reaches values of 0.1nc , 1nc , and 10nc at the positions x = 90, 142, and 150 µ m, respectively.
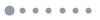
Fig. 13. Evolution of the spatially resolved energy distribution of electrons with time (E is the electron kinetic energy). At 1 ps, the dotted black line represents the position of the laser beam center. As in Fig. 12 , the laser propagates from left to right. The preplasma is distributed over the interval from 0 to 150 µ m, and the dense plasma layer is in the interval from 150 to 160 µ m.
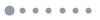
Fig. 14. Distribution of the electrons with x > 240 µ m at 2 ps: energy spectra for selected electrons with angles of 13.5° ± 1° and 58.5° ± 1°, together with the corresponding exponential fits.
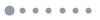
Fig. 15. Proton spectra obtained at t = 3.1 ps for protons within angles of emission smaller than 10° (nearly all the protons are included) and smaller than 1°.
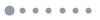
Fig. 16. Density of fast ions obtained at the end of the simulation (3.1 ps), just before that the fastest protons reach the boundary of the simulation box. (a) Proton and (b) C6+ density maps. (c) Longitudinal and (d) transverse electric fields, averaged over the laser period 2π/ω0.
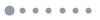
Fig. 17. Data obtained at the end of the simulation (3.1 ps). (a) Distribution of proton energy (>8 MeV) as a function of position ( x , y ) . A clear correlation between the energy and the position is obtained. (b) Angular distribution of protons.
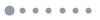
Fig. 18. Comparison of the proton spectra simulated with CALDER-CIRC and the experimental data for shot #176. In CALDER-CIRC, the protons emitted in a cone with a half-angle of 10° were used to compute the average spectrum over the corresponding solid angle.
|
Table 1. Summary of hot-electron measurements using the SESAME diagnostics.
|
Table 2. Estimation of ejected hot-electron charge using Eq. (1) and the value of the hot-electron velocity corresponding to the electron temperature obtained from the electron spectra shown in Fig. 5 .
|
Table 3. Comparison of our results related to proton emission on PETAL with results obtained on other high-energy, high-intensity laser facilities.
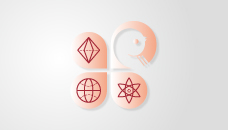
Set citation alerts for the article
Please enter your email address