X. H. Yang, C. Ren, H. Xu, Y. Y. Ma, F. Q. Shao, "Transport of ultraintense laser-driven relativistic electrons in dielectric targets," High Power Laser Sci. Eng. 8, 010000e2 (2020)

Search by keywords or author
- High Power Laser Science and Engineering
- Vol. 8, Issue 1, 010000e2 (2020)
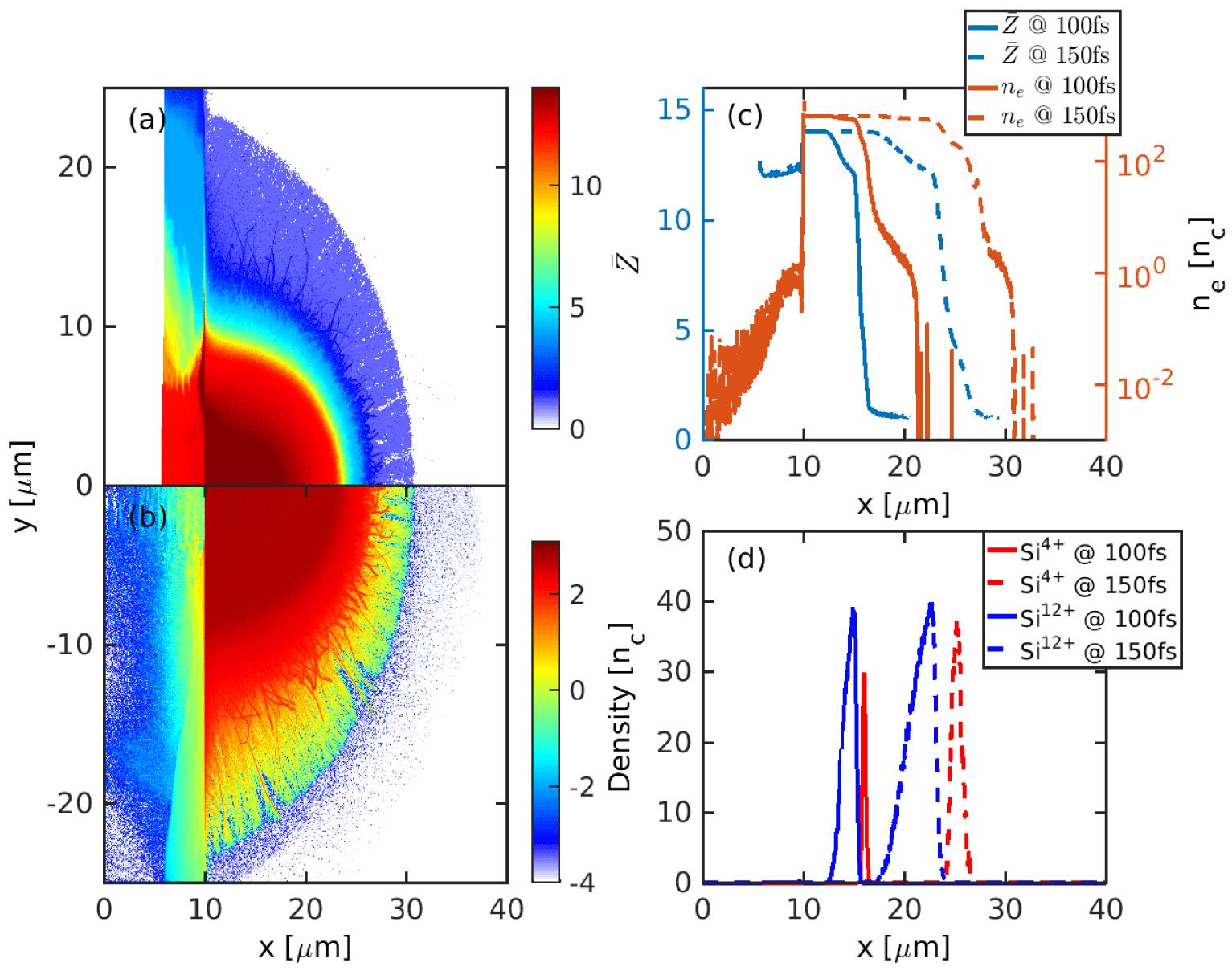
Fig. 1. Distributions of average ionization degree ($\bar{Z}$ ) (a) and $\log _{10}$ of electron density ($n_{e}$ ) (b) at $t=150~\text{fs}$ . Profiles of $\bar{Z}$ and $n_{e}$ (c) and density of $\text{Si}^{4+}$ and $\text{Si}^{12+}$ (d) along the laser propagation axis at $t=100~\text{fs}$ and 150 fs, which are averaged over one wavelength around $y=0$ . Both the electron and ion densities are in units of $n_{c}$ here and in other figures.
![Distributions of the quasi-static magnetic field ($B_{z}$) [(a) and (b)], the longitudinal electrostatic field ($E_{x}$) [(c) and (d)] and the transverse electrostatic field ($E_{y}$) [(e) and (f)] at $t=100~\text{fs}$ [(a), (c) and (e)] and 150 fs [(b), (d), and (f)]. The fields are averaged over two laser cycles and the fields in front of the solid target ($z) are not shown for clarity. The magnetic field and electric field are in units of tesla and $\text{V}/\text{m}$, respectively.](/richHtml/hpl/2020/8/1/010000e2/img_2.png)
Fig. 2. Distributions of the quasi-static magnetic field ($B_{z}$ ) [(a) and (b)], the longitudinal electrostatic field ($E_{x}$ ) [(c) and (d)] and the transverse electrostatic field ($E_{y}$ ) [(e) and (f)] at $t=100~\text{fs}$ [(a), (c) and (e)] and 150 fs [(b), (d), and (f)]. The fields are averaged over two laser cycles and the fields in front of the solid target ($z<10~\unicode[STIX]{x03BC}\text{m}$ ) are not shown for clarity. The magnetic field and electric field are in units of tesla and $\text{V}/\text{m}$ , respectively.
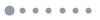
Fig. 3. The energy spectrum of the electrons (a) and the distribution of the electron longitudinal momentum ($P_{x}$ ) along the laser axis at $t=150~\text{fs}$ (b).
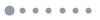
Fig. 4. Current density distributions for the relativistic electrons (kinetic energy $K_{e}\geqslant 50~\text{keV}$ ) (a) and cold electrons ($K_{e}\leqslant 10~\text{keV}$ ) (b) at $t=150~\text{fs}$ . The current is in units of $en_{c}c$ .
![Fast Fourier transform of $E_{x}$ (a) and $E_{y}$ (b) behind the ionization front (i.e., $23~\unicode[STIX]{x03BC}\text{m}) at $t=150~\text{fs}$. $k_{0}$ is the wave number of the laser pulse.](/Images/icon/loading.gif)
Fig. 5. Fast Fourier transform of $E_{x}$ (a) and $E_{y}$ (b) behind the ionization front (i.e., $23~\unicode[STIX]{x03BC}\text{m}) at $t=150~\text{fs}$ . $k_{0}$ is the wave number of the laser pulse.
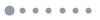
Fig. 6. Profiles of the average ionization degree $\bar{Z}$ (a) and electron density $n_{e}$ (b) along the laser propagation axis (averaged over one wavelength near $y=0$ ) at $t=150~\text{fs}$ for laser intensities of $5\times 10^{18}~\text{W}/\text{cm}^{2}$ , $1\times 10^{19}~\text{W}/\text{cm}^{2}$ , $5\times 10^{19}~\text{W}/~\text{cm}^{2}$ and $1\times 10^{20}~\text{W}/\text{cm}^{2}$ .
![The velocity of ionization wave as a function of laser intensity in the dielectric target, where 1D theory denotes the results from Equation (4); UNR and LULI denote the experimental results from Refs. [12, 14], respectively.](/Images/icon/loading.gif)
Fig. 7. The velocity of ionization wave as a function of laser intensity in the dielectric target, where 1D theory denotes the results from Equation (4 ); UNR and LULI denote the experimental results from Refs. [12, 14], respectively.
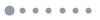
Fig. 8. The energy spectrum of electrons (a) and distribution of the electron longitudinal momentum ($P_{x}$ ) along the laser axis at $t=150~\text{fs}$ (b) for the case with a $\text{Si}^{3+}$ target and without the ionization process. The electron energy spectrum for the ionization case is also presented for comparison.
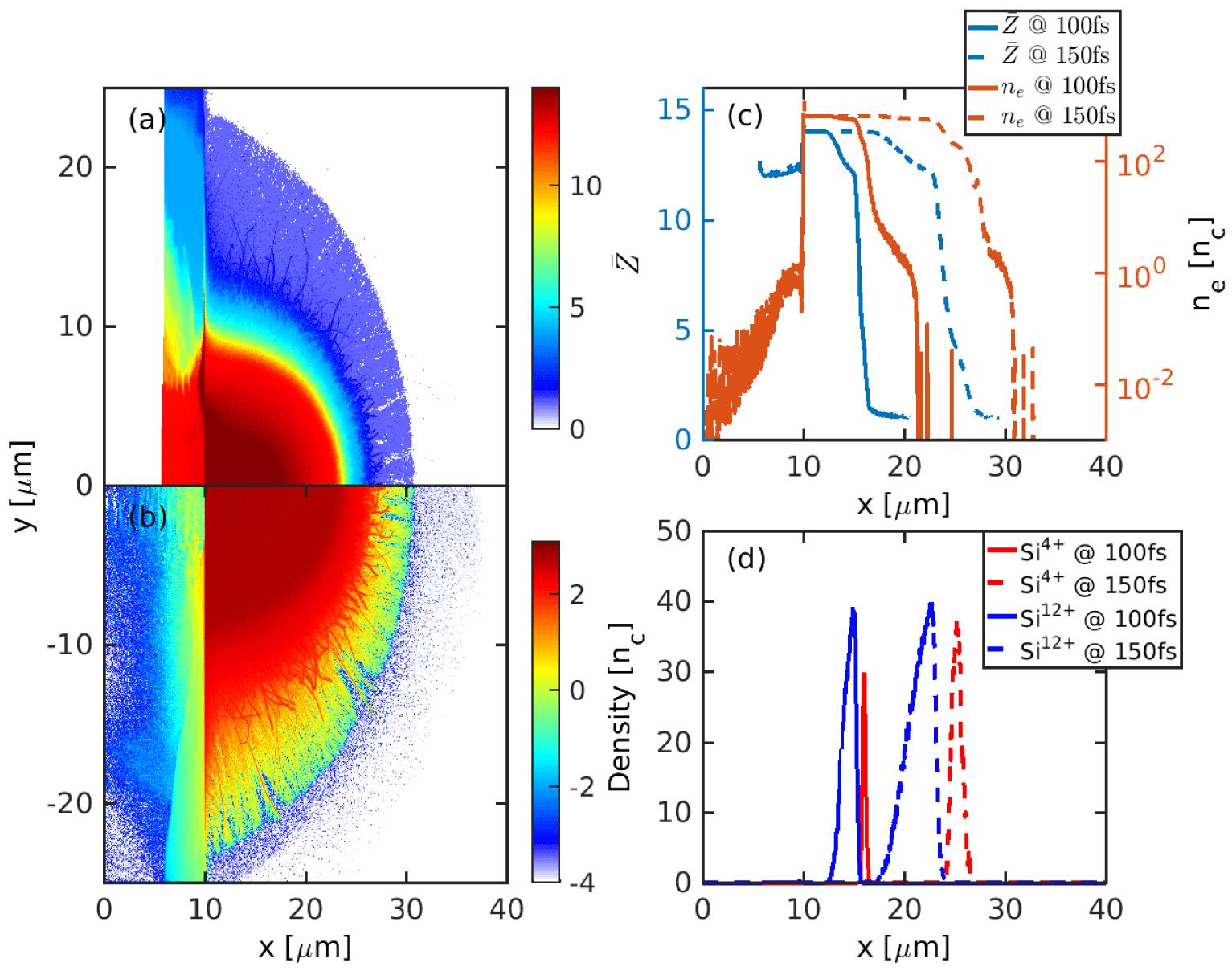
Set citation alerts for the article
Please enter your email address