Hervé C. Lefèvre. Comments about dispersion of light waves[J]. Journal of the European Optical Society-Rapid Publications, 2022, 18(1): 2022001

Search by keywords or author
Journals >Journal of the European Optical Society-Rapid Publications >Volume 18 >Issue 1 >Page 2022001 > Article
- Journal of the European Optical Society-Rapid Publications
- Vol. 18, Issue 1, 2022001 (2022)
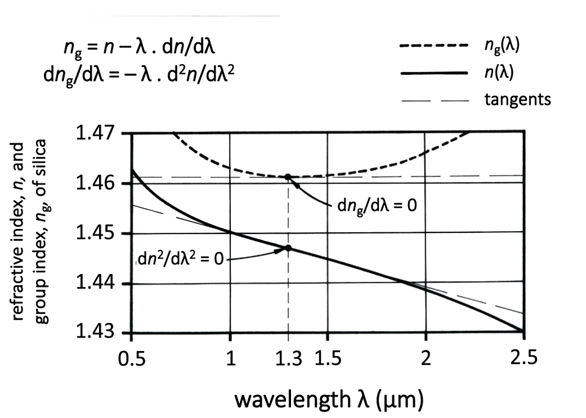
Fig. 1. Refractive (or phase) index n (solid line curve), and group index ng (dashed line curve) of silica, as a function of the wavelength λ in a vacuum. At 1.3 μm, there is no group velocity dispersion since ng is constant, i.e., dng/dλ is null. This is the basic cause; the fact that d2n/dλ2 = 0 at 1.3 μm is just a consequence of dng/dλ = −λ · d2n/dλ2.
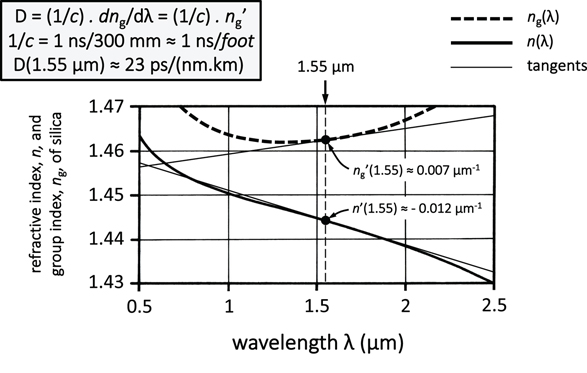
Fig. 2. Refractive (or phase) index n (solid line curve), and group index ng (dashed line curve) of silica, as a function of the wavelength λ in a vacuum. At 1.55 μm, the slope of the tangent to the curve n(λ), i.e., dn/dλ or n′(λ), equates minus 0.012 μm−1, and gives the chromatic, or first-order, dispersion; the one to the curve ng(λ), i.e., dng/dλ or n′g(λ), equates plus 0.007 μm−1, and gives the group velocity dispersion, or second-order dispersion. Since 1/c = 1 ns/300 mm, the dispersion parameter D(1.55 μm) = (1/c) · n′g(1.55 μm) equates + 23 ps/(nm km).
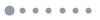
Fig. 3. Refractive (or phase) index n(λ) (solid line), and group index ng(λ) (dashed line), in the theoretical case of a material without any group dispersion. The straight line representing n(λ) crosses the ordinate axis corresponding to λ = 0, at the constant value of ng(λ) = n(0).
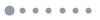
Fig. 4. Refractive (or phase) index n(λ) (solid line curve), with group dispersion. The tangent T0(λ) to the curve n(λ) at λ0 crosses the ordinate axis corresponding to λ = 0, at the value ng(λ0) of the group index for λ0.
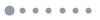
Fig. 5. Refractive (or phase) index n(λ) (solid line curve), and group index ng(λ) (dashed line curve) of silica. The tangent to the curve n(λ), at λ0, crosses the extended ordinate axis, where λ = 0 μm, at the value ng(λ0) of the group index for λ0, as shown for λ0 equal to 0.85 μm, or to 1.3 μm.
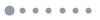
Fig. 6. Effective index neff of the fundamental mode, n1 being the refractive index of the core, and n2 being the one of the cladding: (a) classical representation, as a function of the normalized spatial frequency λc/λ, in a vacuum; (b) inverted representation as a function of the normalized wavelength λ/λc, in a vacuum.
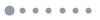
Fig. 7. Geometrical construction to derive the effective group index ng-eff(λ/λc) of the fundamental mode (solid line curve) from its effective phase index neff(λ/λc) (dashed line curve). The tangent to neff(λ/λc) crosses the ordinate axis at the value of the corresponding effective group index ng-eff(λ/λc), as shown for λ = 1.25 λc. One sees easily that ng-eff is about equal to the refractive index of the core n1, in the practical domain of use of a single-mode fiber, i.e., λc < λ < 1.5 λc; above 1.5 λc, the mode starts to widen a lot and the curvature loss increases drastically.
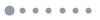
Fig. 8. Two possible geometrical constructions for relating group index ng to phase index n: (a) with the dependence in wavelength λ, i.e., the spatial period of the wave, the tangent T0(λ) to the phase index curve crosses the ordinate axis at the value ng(λ0) of the group index; (b) with the dependence in angular frequency ω, a double-slope line DS0(ω) is drawn from where the tangent T0(ω) to the phase index curve crosses the ordinate axis, and the group index ng(ω0) equates DS0(ω0).
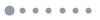
Fig. 9. Geometrical construction relating the effective group index ng-eff to the effective index neff of the fundamental mode of a fiber, with the dependence in normalized spatial frequency λc/λ. A double-slope line is drawn from where the tangent to the effective index curve crosses the ordinate axis, and the group index ng(λc/λ0) equates DS0(λc/λ0), as shown for λc/λ0 = 0.8, i.e., for λ0 = 1.25 λc. As in Figure 7 , one easily sees that ng-eff is about equal to the refractive index of the core n1, in the practical domain of use of a single-mode fiber, i.e., λc < λ < 1.5 λc, or 0.67 < λc/λ < 1.
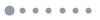
Fig. 10. Geometrical construction relating group birefringence Bg to phase birefringence B(λ) (solid line curve); the tangent T0(λ) to the curve B(λ) at λ0, crosses the ordinate axis corresponding to λ = 0, at the value Bg(λ0) of the group birefringence for λ0. This figure is obviously derived from Figure 4 .
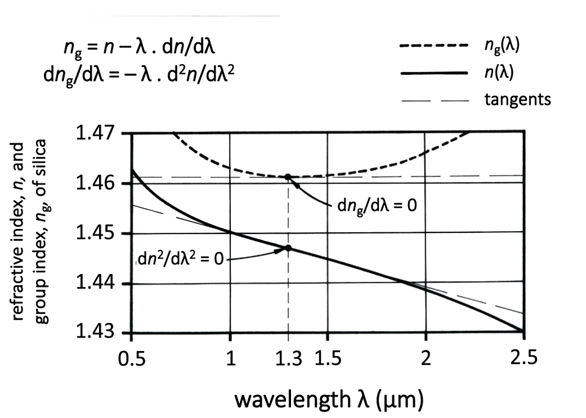
Set citation alerts for the article
Please enter your email address