Ting Fu, Yufei Wang, Xueyou Wang, Jingxuan Chen, Xuyan Zhou, Wanhua Zheng. Microstructure Lasers Based on Parity-Time Symmetry and Supersymmetry[J]. Chinese Journal of Lasers, 2021, 48(12): 1201005

Search by keywords or author
- Chinese Journal of Lasers
- Vol. 48, Issue 12, 1201005 (2021)
![Theory of lateral PT symmetry[46,81]. (a) Schematic of laterally PT-symmetric double waveguide arrays; (b)(c) the corresponding complex propagation constant versus the imaginary part of the complex refractive index of the gain waveguide; (d)(e) mode field distributions of fundamental supermode and high-order supermode in the PT-symmetric waveguide, respectively. White solid line is the real part of the complex refractive index, white dashed line is the imaginary part of the complex refractive index, and yellow line is the electric field intensities](/richHtml/zgjg/2021/48/12/1201005/img_1.jpg)
Fig. 1. Theory of lateral PT symmetry[46,81]. (a) Schematic of laterally PT-symmetric double waveguide arrays; (b)(c) the corresponding complex propagation constant versus the imaginary part of the complex refractive index of the gain waveguide; (d)(e) mode field distributions of fundamental supermode and high-order supermode in the PT-symmetric waveguide, respectively. White solid line is the real part of the complex refractive index, white dashed line is the imaginary part of the complex refractive index, and yellow line is the electric field intensities
![Theory of longitudinal PT symmetry[43-44]. (a)--(c) Schematics of the longitudinally PT-symmetric two-port optical system, the traditional laser cavity, and the longitudinally PT-symmetric distributed feedback waveguide, respectively; (d)(e) behavior of the overall reflection/transmission coefficient versus angular frequency detuning for the longitudinally PT-symmetric two-port optical system and the longitudinally PT-symmetric distributed feedback waveguide, respectively, solid line is the two-port coherent input excitation satisfying d/a=M21, dashed line is the two-port incoherent input excitation with the averaged random phase, and dotted line is the single-port input excitation; (f) distributions of the poles and the zeros in the complex plane and log of transmittance for one longitudinally PT-symmetric optical system](/richHtml/zgjg/2021/48/12/1201005/img_2.jpg)
Fig. 2. Theory of longitudinal PT symmetry[43-44]. (a)--(c) Schematics of the longitudinally PT-symmetric two-port optical system, the traditional laser cavity, and the longitudinally PT-symmetric distributed feedback waveguide, respectively; (d)(e) behavior of the overall reflection/transmission coefficient versus angular frequency detuning for the longitudinally PT-symmetric two-port optical system and the longitudinally PT-symmetric distributed feedback waveguide, respectively, solid line is the two-port coherent input excitation satisfying d/a=M21, dashed line is the two-port incoherent input excitation with the averaged random phase, and dotted line is the single-port input excitation; (f) distributions of the poles and the zeros in the complex plane and log of transmittance for one longitudinally PT-symmetric optical system
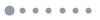
Fig. 3. Theory of supersymmetric transformation. (a) Schematic of supersymmetric transformation; (b) near field distribution and far field distribution of the supersymmetric laser array with five waveguide elements calculated by finite element method
![Laterally PT-symmetric micro-structure lasers[49,51]. (a)--(c) Mode field distributions of the fundamental mode, the first order mode, and the second order mode in the cross-section of the single micro-ring resonator, respectively; (d)--(f) mode field distributions of the corresponding supermodes in the PT-symmetric double ring resonators; (g) coupling coefficients of different modes versus distance between the two rings; (h)(i) optical spectra of the single ring laser and the PT-symmetric double ring laser under different optical pumping powers, respectively; (j) schematic of the laterally PT-symmetric double broad area coupled single lateral-mode laser; (k)--(m) far field distributions of the laser under different injected currents in the lossy waveguide and the insets are the corresponding near field distributions around the cavity mirror](/Images/icon/loading.gif)
Fig. 4. Laterally PT-symmetric micro-structure lasers[49,51]. (a)--(c) Mode field distributions of the fundamental mode, the first order mode, and the second order mode in the cross-section of the single micro-ring resonator, respectively; (d)--(f) mode field distributions of the corresponding supermodes in the PT-symmetric double ring resonators; (g) coupling coefficients of different modes versus distance between the two rings; (h)(i) optical spectra of the single ring laser and the PT-symmetric double ring laser under different optical pumping powers, respectively; (j) schematic of the laterally PT-symmetric double broad area coupled single lateral-mode laser; (k)--(m) far field distributions of the laser under different injected currents in the lossy waveguide and the insets are the corresponding near field distributions around the cavity mirror
![Electrically injected PT-symmetric micro-structure lasers[52,82]. (a)--(c) Scanning electron microscope images of the electrically injected double ring laser; (d)--(f) optical spectra of the double ring lasers with the two rings uniformly pumped, the left ring pumped only, and the right ring pumped only, respectively, and the insets are the corresponding light intensity profiles; (g) microscopic photo of electrically injected PT-symmetric coherently coupled VCSEL array; (h)(i) near field distributions and far field distributions of the laser under different injected currents Ib](/Images/icon/loading.gif)
Fig. 5. Electrically injected PT-symmetric micro-structure lasers[52,82]. (a)--(c) Scanning electron microscope images of the electrically injected double ring laser; (d)--(f) optical spectra of the double ring lasers with the two rings uniformly pumped, the left ring pumped only, and the right ring pumped only, respectively, and the insets are the corresponding light intensity profiles; (g) microscopic photo of electrically injected PT-symmetric coherently coupled VCSEL array; (h)(i) near field distributions and far field distributions of the laser under different injected currents Ib
![PT-symmetric double ring laser with embedded S waveguide[85]. (a) Scanning electron microscope of the laser; (b) schematic of the unidirectional light propagation in the laser; (c)(e) camera image and optical spectrum of the laser within the PT-symmetric phase, respectively; (d)(f) camera image and optical spectrum of the laser within the broken PT-symmetric phase, respectively](/Images/icon/loading.gif)
Fig. 6. PT-symmetric double ring laser with embedded S waveguide[85]. (a) Scanning electron microscope of the laser; (b) schematic of the unidirectional light propagation in the laser; (c)(e) camera image and optical spectrum of the laser within the PT-symmetric phase, respectively; (d)(f) camera image and optical spectrum of the laser within the broken PT-symmetric phase, respectively
![Longitudinally PT-symmetric single mode lasers[38,54]. (a) Schematic of longitudinally PT-symmetric micro-ring laser modulated by gain and loss; (b) optical spectra of the laser under different optical pumping intensities; (c) scanning electron microscope of the PT-symmetric CPA-laser; (d) enlarged grating area of the CPA-laser; (e) optical spectrum of the CPA-laser; (f) light-light curve of the CPA-laser; (g) global reflection/transmission coefficient versus wavelength, and red points represent coherent amplification and blue points represent coherent absorption](/Images/icon/loading.gif)
Fig. 7. Longitudinally PT-symmetric single mode lasers[38,54]. (a) Schematic of longitudinally PT-symmetric micro-ring laser modulated by gain and loss; (b) optical spectra of the laser under different optical pumping intensities; (c) scanning electron microscope of the PT-symmetric CPA-laser; (d) enlarged grating area of the CPA-laser; (e) optical spectrum of the CPA-laser; (f) light-light curve of the CPA-laser; (g) global reflection/transmission coefficient versus wavelength, and red points represent coherent amplification and blue points represent coherent absorption
![Phenomena of doubled mode spacings in longitudinally PT-symmetric lasers[37,57]. (a) Schematic of the uniformly optically pumped stripe laser; (b) schematic of the partially optically pumped PT-symmetric stripe laser; (c) optical spectrum of the uniformly optically pumped stripe laser; (d) optical spectrum of the partially optically pumped PT-symmetric stripe laser; (e) schematic of the electrically injected PT-symmetric laser; (f) optical spectra of the laser under different injected currents](/Images/icon/loading.gif)
Fig. 8. Phenomena of doubled mode spacings in longitudinally PT-symmetric lasers[37,57]. (a) Schematic of the uniformly optically pumped stripe laser; (b) schematic of the partially optically pumped PT-symmetric stripe laser; (c) optical spectrum of the uniformly optically pumped stripe laser; (d) optical spectrum of the partially optically pumped PT-symmetric stripe laser; (e) schematic of the electrically injected PT-symmetric laser; (f) optical spectra of the laser under different injected currents
![Longitudinally PT-symmetric OAM lasers[55-56]. (a)(b) Scanning electron microscope of the PT-symmetric OAM micro-ring laser modulated by complex refractive index and corresponding enlarged picture of the ring area; (c) optical spectra of the laser under different optical pumping intensities; (d) light-light curve of the laser; (e) far field distribution of the laser; (f) off-center self-interference pattern of the laser; (g) scanning electron microscope of the PT-symmetric tunable vortex laser; (h) enlarged picture of ring area; (i)--(k) simulated phase distributions of the far fields, off-center self-interference patterns, and optical spectra with OAM equal to -2, -1, 0, 1, 2, respectively](/Images/icon/loading.gif)
Fig. 9. Longitudinally PT-symmetric OAM lasers[55-56]. (a)(b) Scanning electron microscope of the PT-symmetric OAM micro-ring laser modulated by complex refractive index and corresponding enlarged picture of the ring area; (c) optical spectra of the laser under different optical pumping intensities; (d) light-light curve of the laser; (e) far field distribution of the laser; (f) off-center self-interference pattern of the laser; (g) scanning electron microscope of the PT-symmetric tunable vortex laser; (h) enlarged picture of ring area; (i)--(k) simulated phase distributions of the far fields, off-center self-interference patterns, and optical spectra with OAM equal to -2, -1, 0, 1, 2, respectively
![Optically pumped SUSY laser arrays[77-78]. (a) Scanning electron microscope of the SUSY laser array; (b) mode field distributions of different order supermodes of the laser array; (c)--(e) optical spectra of the single ridge laser, the traditional laser array, and the SUSY laser array, respectively; (f)--(h) corresponding lateral far field distributions of the three kinds of lasers; (i) schematic of the second-order SUSY micro-ring laser array; (j) mode field distributions of the modes before and after the main array coupled with the superpartners; (k) optical spectra of the laser array under different optical pumping intensities](/Images/icon/loading.gif)
Fig. 10. Optically pumped SUSY laser arrays[77-78]. (a) Scanning electron microscope of the SUSY laser array; (b) mode field distributions of different order supermodes of the laser array; (c)--(e) optical spectra of the single ridge laser, the traditional laser array, and the SUSY laser array, respectively; (f)--(h) corresponding lateral far field distributions of the three kinds of lasers; (i) schematic of the second-order SUSY micro-ring laser array; (j) mode field distributions of the modes before and after the main array coupled with the superpartners; (k) optical spectra of the laser array under different optical pumping intensities
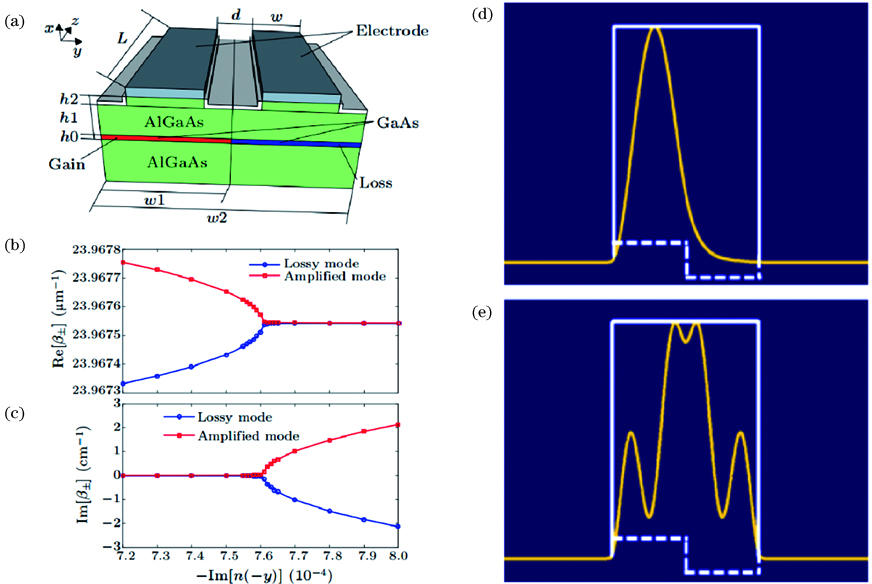
Set citation alerts for the article
Please enter your email address