Bingshuang Yao, Xiaofei Zang, Zhen Li, Lin Chen, Jingya Xie, Yiming Zhu, Songlin Zhuang, "Dual-layered metasurfaces for asymmetric focusing," Photonics Res. 8, 830 (2020)

Search by keywords or author
- Photonics Research
- Vol. 8, Issue 6, 830 (2020)
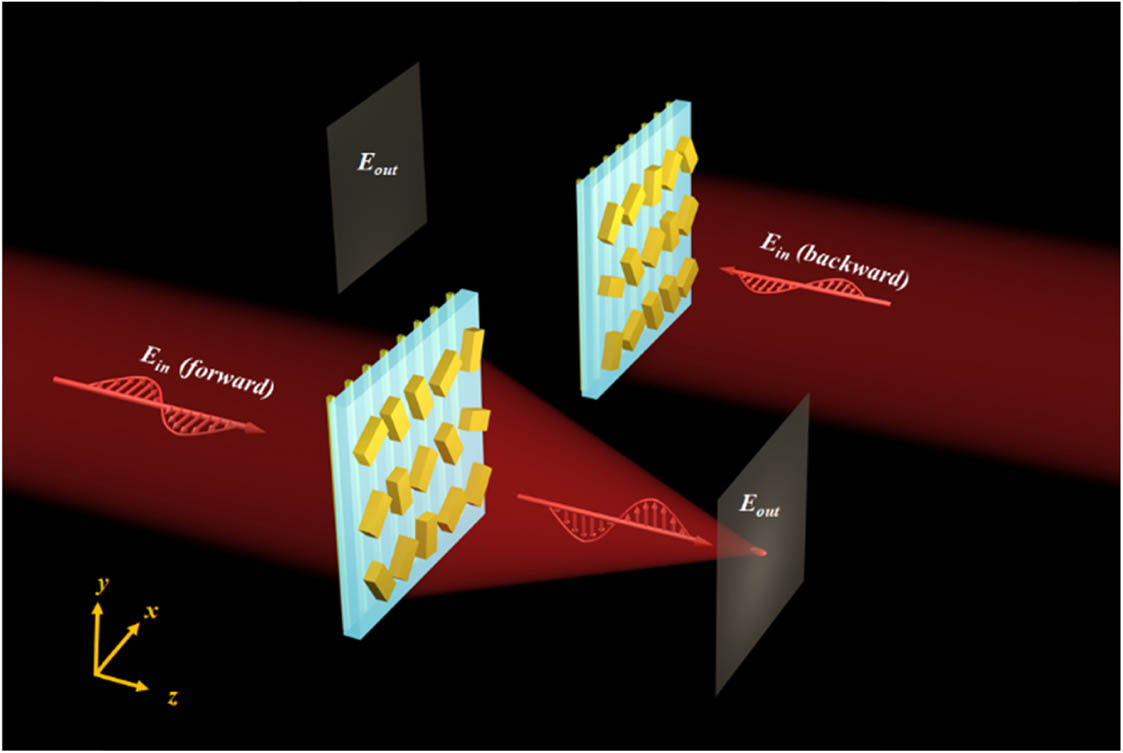
Fig. 1. Schematic of asymmetric focusing. Under the illumination of x -polarized THz waves in the forward direction, a y -polarized focal spot is observed, while the focal spot is not generated for backward x -polarized incidence.
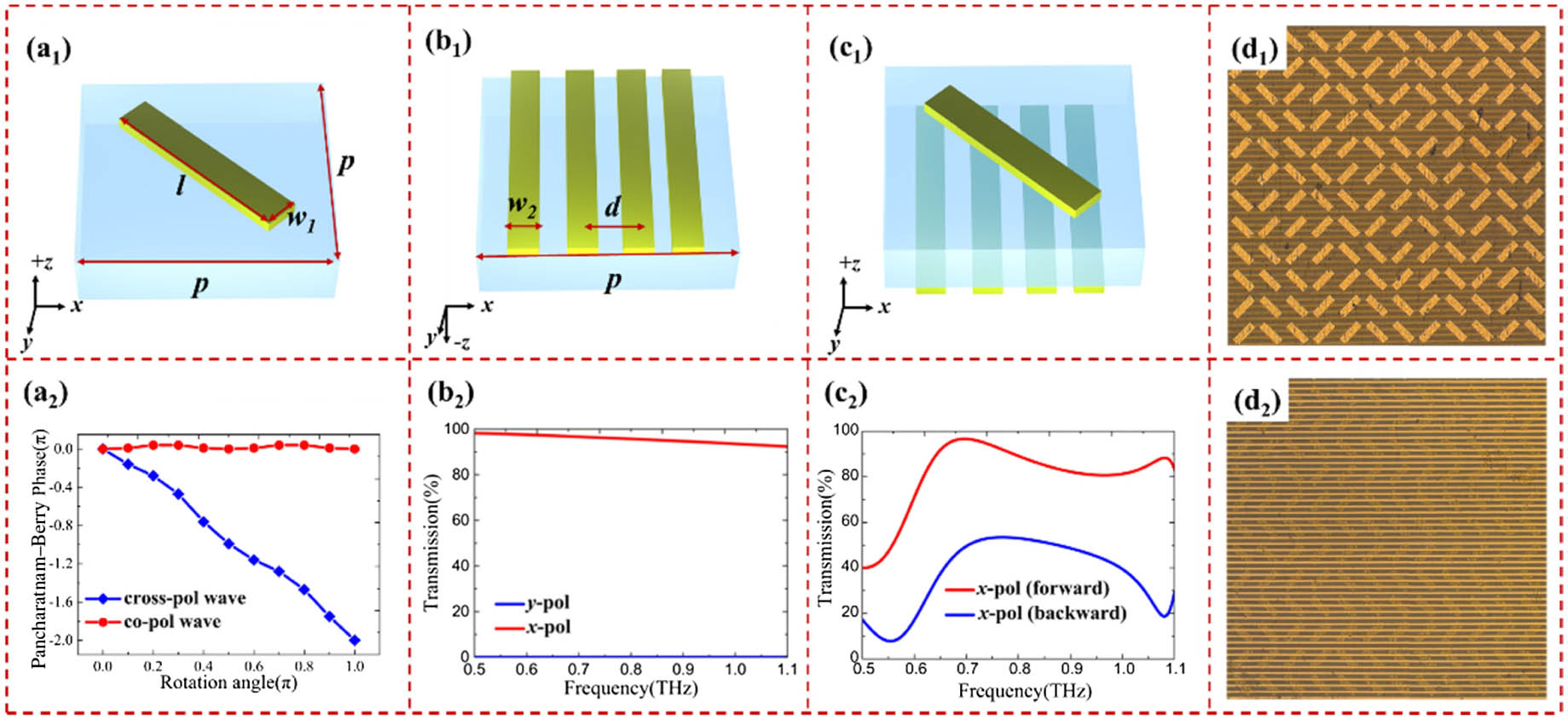
Fig. 2. Design of dual-layered metasurfaces: (a1) and (a2) schematic and the corresponding geometric phase of the microrod; (b1) and (b2) schematic and the corresponding transmission spectra of the metallic gratings; (c1) and (c2) schematic and the corresponding transmission spectra of the metasurface combined with metallic gratings; (d1) and (d2) optical images of the metasurface and metallic gratings.
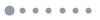
Fig. 3. (a1)–(f1) Numerical simulation of electric field distributions in the x − z plane under the illumination of x -polarized THz waves in the forward/backward direction at 0.6, 0.85, and 1.1 THz; (a2)–(f2) the corresponding electric field distributions in the x − y plane.
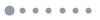
Fig. 4. (a1)–(f1) The measured electric field distributions in the x − z plane under the illumination of x -polarized THz waves in the forward/backward direction at 0.6, 0.85, 1.1 THz; (a2)–(f2) the corresponding electric field distributions in the x − y plane.
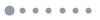
Fig. 5. Numerical simulation of asymmetric transmission with (a) and (b) longitudinal and (c) and (d) transversal multiple focal spots.
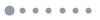
Fig. 6. (a1)–(c1) Schematics of a microrod, metallic gratings, and a unit cell of the directional device. The intersection angle between the long axis of the microrod and the x axis is 45°, while the long axis of gratings is along the x axis. (a2)–(a4) The co-polarized/cross-polarized/total transmission and reflection of the microrod under the illumination of linearly polarized THz waves. (b2)–(b4) The co-polarized/cross-polarized/total transmission and reflection of the metallic gratings under the illumination of linearly polarized THz waves. (c2)–(c4) The co-polarized/cross-polarized/total transmission and reflection of the unit cell of the directional device under the illumination of linearly polarized THz waves. T i j ( R i j ) is the transmission (reflection) of the i -polarized THz waves under the illumination of j -polarized THz waves ( i , j = x , y ) . T i ( R i , i = x , y ) is the total transmission (total reflection) under the illumination of i -polarized THz waves.
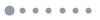
Fig. 7. (a1)–(f1) Numerical simulation of electric field distributions in the x − z plane under the illumination of y -polarized THz waves in the forward direction at 0.6, 0.85, and 1.1 THz; (a2)–(f2) the calculated electric field distributions for backward incidence.
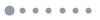
Fig. 8. The calculated and measured electric field distributions in the x − z plane under the illumination of the x -polarized THz waves from the (a1)–(f1) forward and (a2)–(f2) backward directions at 0.6, 0.85, and 1.1 THz.
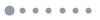
Fig. 9. The calculated electric field distributions in the x − z plane under the illumination of the x -polarized THz waves in the (a1), (b1) forward and (a2), (b2) backward directions at 0.85 THz.
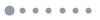
Fig. 10. Calculated efficiency of the directional device under the illumination of x -polarized THz waves in the forward direction.
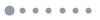
Fig. 11. Schematic of multiple transmissions from the dual-layered metasurfaces.
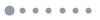
Fig. 12. Comparison of the numerical (blue curves) and experimental (red curves) focusing properties: (a)–(c) the corresponding electric field distributions at x = 0 in the focal plane.
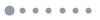
Fig. 13. Schematics for the extinction ratio defined as (a) T E y / T E x and (b) T E y 1 / T E y 2 .
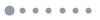
Fig. 14. (a1)–(c1) Calculated electric field ( | E y | 2 ) distributions in the x − z plane under the illumination of x -polarized THz waves (with different incident angles) in the forward directions at 0.85 THz; (a2)–(c2) the calculated electric field distributions for backward incidence. Insets show the schematics for the incident THz waves with a tilted wavefront.
|
Table 1. Size of the Focal Point
|
Table 2. Comparison Between the Diffraction Limit in Theory and the FWHM of the Focal Spots
|
Table 3. Extinction Ratio Between and
|
Table 4. Extinction Ratio Between the Forward and Backward Directions
|
Table 5. Extinction Ratio for the Directional Device with Two Focal Spots
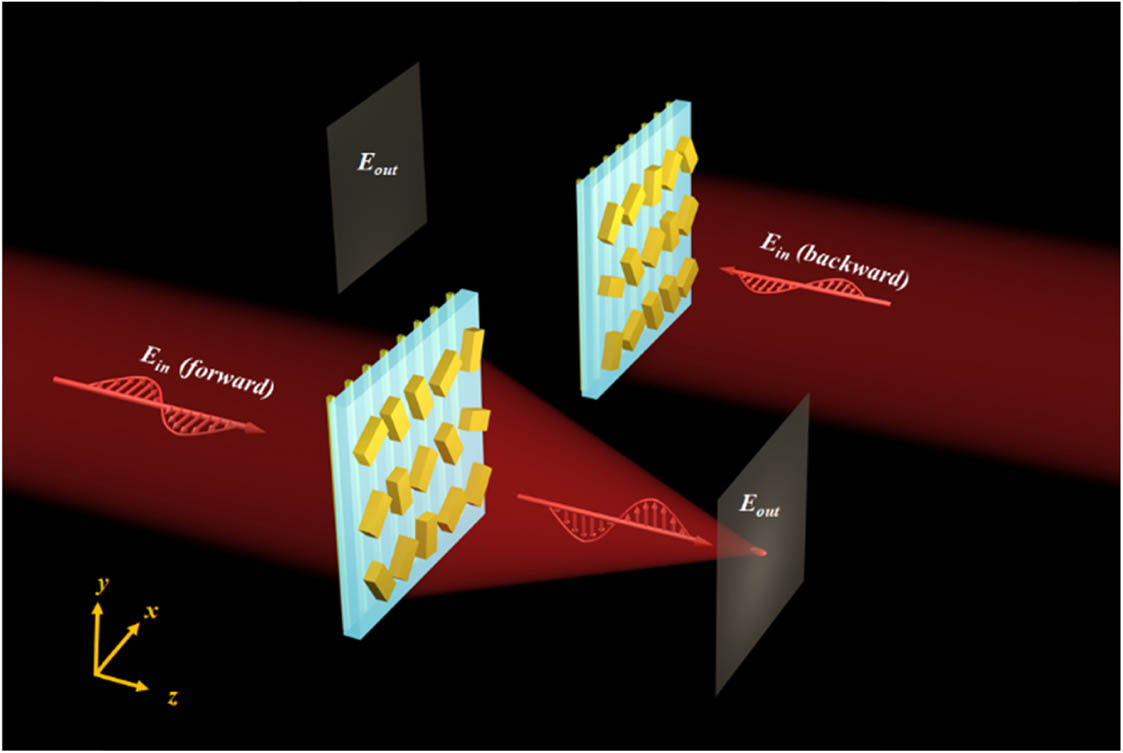
Set citation alerts for the article
Please enter your email address