Author Affiliations
1Institute of Microelectronics, Chinese Academy of Sciences, Beijing 100029, China2University of Chinese Academy of Sciences, Beijing 100049, China3Suzhou Institute of Nano-Tech and Nano-Bionics, Chinese Academy of Sciences, Suzhou 215123, Chinashow less
Abstract
In this letter, an enhancement-mode (E-mode) GaN p-channel field-effect transistor (p-FET) with a high current density of ?4.9 mA/mm based on a O3-Al2O3/HfO2 (5/15 nm) stacked gate dielectric was demonstrated on a p++-GaN/p-GaN/AlN/AlGaN/AlN/GaN/Si heterostructure. Attributed to the p++-GaN capping layer, a good linear ohmic I?V characteristic featuring a low-contact resistivity (ρc) of 1.34 × 10?4 Ω·cm2 was obtained. High gate leakage associated with the HfO2 high-k gate dielectric was effectively blocked by the 5-nm O3-Al2O3 insertion layer grown by atomic layer deposition, contributing to a high ION/IOFF ratio of 6 × 106 and a remarkably reduced subthreshold swing (SS) in the fabricated p-FETs. The proposed structure is compelling for energy-efficient GaN complementary logic (CL) circuits.Introduction
In last few decades, GaN power high-electron-mobility transistors (HEMTs) have become an indispensable core component of power electronics, owing to their high breakdown voltage and fast switching speed[1−6]. To further enhance the reliability and speed of the device, monolithically integrated GaN-based peripheral circuits composed of logic modules that serve as the driving, control, sensing and protection units, are highly desirable. One of the promising technologies, especially for high-temperature operation application, is the GaN-based CMOS platform, which delivers extremely low power consumption[7]. The key issue of GaN-based CMOS technology is the low current density of the GaN p-FETs, which ascribe to the high activation energy (~170 meV) of Mg doping, low hole mobility and the difficulty in forming ohmic contact due to the p-GaN deep valance bands[8−10]. To improve the conduction characteristic of the GaN p-channel device, polarization-enhanced epitaxy technology was adopted to induce high-density two-dimensional hole gas (2DHG), which can effectively increase carrier concentration[11−14]. At the same time, in order to achieve the enhancement mode (E-mode), a partial recessed p-GaN channel in the gate region is needed[15−22].
As the core component of the pull-up device in GaN-based CMOS logic circuit topology, the p-FETs with a low subthreshold slope (SS) are beneficial for achieving lower power consumption of the logic circuit due to the operating voltage reduction[23]. A high-k gate dielectric is a facile method that reduces the SS of the devices. Among all the high-k dielectrics, HfO2 is favorable due to its large dielectric constant (~25) and perfect compatibility with CMOS fabrication processes[24]. Therefore, HfO2 is selected as the high-k dielectric material for our fabricated GaN p-FETs.
In this work, E-mode GaN p-FETs with O3-Al2O3/HfO2-stacked gate dielectric are fabricated on a p++-GaN/p-GaN/AlN/AlGaN/AlN/GaN heterostructure grown on a Si substrate. The O3-Al2O3 insertion layer effectively improves the voltage blocking of the stack dielectric and reduces the leakage current. Meanwhile, the introduction of HfO2 reduces the SS of GaN p-FETs from 161 mV/dec to 107 mV/dec, which significantly improves the current performance of the GaN p-FETs. The improved subthreshold performance of the p-FETs enables high ON-OFF current ratio with low operating voltage, which is beneficial for reducing the power consumption of GaN-based CMOS logic driver circuits.
Epitaxial structure and dielectric leakage characterization
Fig. 1(a) shows the cross-sectional schematic of the fabricated metal–oxide–semiconductor (MOS) device for dielectric leakage characterization. The employed p++-GaN/p-GaN/AlN/AlGaN/AlN/GaN heterostructure was grown by metal–organic chemical vapor deposition (MOCVD) on the Si substrate. It consists of a ~10-nm p++-GaN capping layer (Mg: 2 × 1020 cm−3), a ~85-nm p-GaN layer (Mg: (4–6) × 1019 cm−3), a ~2-nm AlN polarization enhancement layer, a ~3-nm Al0.25Ga0.75N ultrathin barrier layer (UTB), a ~1-nm AlN interface enhancement layer, a 300-nm unintentionally doped GaN n-channel layer and a 3.6-µm (Al)GaN high-resistivity buffer layer with C doping. The 3-nm UTB-Al0.25Ga0.75N layer is intended for a AlGaN-recess-free E-mode n-FETs for p/n-FETs integration[25]. The hole sheet density and mobility were measured to be 2.3 × 1013 cm−2 and 11.5 cm2/(V·s) respectively by Van der Pauw Hall measurement. The gate dielectrics for comparison are a 20-nm HfO2, 20-nm O3-Al2O3 and an O3-Al2O3/HfO2 (5/15 nm) stack, both of which are grown by atomic layer deposition (ALD).
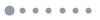
Figure 1.(Color online) (a) Cross-sectional schematic and (b) current density−voltage curves of the MOS device on the p++-GaN/p-GaN/AlN/AlGaN/AlN/GaN heterostructure.
The leakage characteristic of different dielectrics is shown in Fig. 1(b). The 20-nm HfO2 shows a lower breakdown voltage and higher leakage current density than that of the 20-nm O3-Al2O3 due to its lower band gap (HfO2: ~5.8 eV, O3-Al2O3: ~7.1 eV) and valence band offset from p-GaN (p-GaN/HfO2: ~0.3 eV, p-GaN/O3-Al2O3: ~1.7 eV)[26, 27]. The introduction of the 5-nm O3-Al2O3 insertion layer can effectively reduce the leakage of the O3-Al2O3/HfO2 stack and improve its voltage-blocking capability.
Device fabrication and characteristics of E-mode GaN p-FETs
Fig. 2(a) exhibits the cross-sectional schematic of the fabricated E-mode GaN p-FETs. Device fabrication commenced with source/drain definition by photolithography, followed by Ni/Au (50/100 nm) metal stack evaporation. Rapid thermal annealing (RTA) in the air environment at 550 °C for 60 s was performed after lift-off. Thanks to the p++-GaN capping layer, linear I−V curves were obtained. The contact resistance (Rc) of 18.86 Ω·mm (ρc = 1.34 × 10−4 Ω·cm2) and sheet resistance (Rsh) of 2.65 × 104 Ω/sq are determined by the transfer length method (TLM) respectively, as shown in Fig. 2(b). Fig. 2(c) benchmarks the Rc and hole sheet density of the heterostructure used in this work with other similar Ⅲ-nitride heterostructures. The low Rc achieved is comparable to state-of-the-art results.
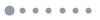
Figure 2.(Color online) (a) Cross-sectional schematic of the fabricate E-mode GaN p-FETs. (b) TLM analysis of the ohmic contact. (c) Benchmarking the Rc and hole sheet density of the fabricated GaN p-FET with some state-of-the-art GaN p-FETs. (d) Depth profile of the gate recess trench measured by the atomic force microscope. The inset figure presents measured resistances as a function of ohmic metal spacing.
The mesa isolation was implemented by the Cl2/BCl3-based inductively coupled plasma-reactive ion etching (ICP-RIE) process to the GaN buffer. A two-step gate etching process was adopted to overcome the decreased OFF-state blocking voltage associated with the p++-GaN capping layer[28]. The remaining p-GaN under the central gate trench is 8 nm as confirmed by atomic force microscopy (AFM), as shown in Fig. 2(d). The two-step gate trench was then subjected to an UV/O3 treatment at 100 °C for 30 min. Prior to the deposition of the gate dielectric, in-situ remote plasma pretreatments (RPP)[29] by using NH3/N2 plasmas applied were conducted. After the source/drain region opening, the GaN p-FET fabrication was completed with an evaporated Ni/Au (40/400 nm) metal stack as the gate metal and probing pad. For comparison, the devices using the 20-nm O3-Al2O3 and the O3-Al2O3/HfO2 (5/15 nm) stack as the gate dielectric are both fabricated. The fabricated GaN p-FETs possess a gate length (LG) of 2 μm, an equivalent gate to source length (LGS) and a gate to drain length (LGD) of 5 μm.
| Vth (V) | Id,max (mA/mm) | ION/IOFF | SS (mV/dec) |
---|
MIT[16] | −0.5 | −45 | 104 | 800 |
Cornell[20] | −0.35 | −10 | 104 | 1027 |
HRL[22] | −0.36 | −1.65 | 106 | 304 |
HKUST[30] | −1.7 | −6.1 | 107 | 230 |
ASU[31] | −0.6 | −0.2 | 5 × 107 | 123 |
This work | −0.8 | −4.9 | 6 × 106 | 107 |
Table 1. Benchmark of GaN-based p-FETs.
Fig. 3(a) shows DC transfer characteristics of the fabricated GaN p-FETs with an O3-Al2O3 and O3-Al2O3/HfO2 stack as the gate dielectric at VDS of –1 V. Since the 8-nm p-GaN layer only remained at the bottom of the gate trench featuring the weakening of the built-in polarization, both of the devices behave as E-modes and exhibit a high ION/IOFF ratio of 6 × 106. Thanks to the high-quality O3-Al2O3 insertion layer, the device with the O3-Al2O3/HfO2 gate stack shows a low gate leakage below 10−6 mA/mm, the same as the device with 20-nm O3-Al2O3 gate dielectric. In addition, a steeper transfer curve is obtained due to the high dielectric constant of HfO2 for the GaN p-FET with the O3-Al2O3/HfO2 gate stack. Its minimum SS is extracted to be 107 mV/dec, which is significantly lower than the 161 mV/dec of the comparison device (see Fig. 3(b)).
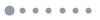
Figure 3.(Color online) (a) DC transfer and (b) SS vs ID plot of fabricated GaN p-FETs with the O3-Al2O3 and O3-Al2O3/HfO2 stack as the gate dielectric.
Fig. 4(a) depicts the output curves of the devices. Owing to the p++-GaN cap layer of the epitaxial structure, an offset voltage, which is usually observed in IDS−VDS curves of p-FETs fabricated on the moderate Mg-doped p-GaN layer[31, 32], is effectively eliminated. The O3-Al2O3/HfO2-stacked device with better channel modulation capability delivers a high-saturation current density of −4.9 mA/mm and an on-resistance (Ron) of 0.70 kΩ·mm at VGS = −10 V. Three-terminal OFF-state characteristics of the fabricated GaN p-FET is plotted in Fig. 4(b). A destructive hard breakdown was observed at −52 and −61 V on the devices with the O3-Al2O3/HfO2 gate stack and the O3-Al2O3 gate dielectric, respectively.
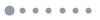
Figure 4.(Color online) (a) DC output and (b) OFF-state characteristics of fabricated GaN p-FETs with the O3-Al2O3 and O3-Al2O3/HfO2 stack as the gate dielectric.
Table 1 benchmarks the fabricated GaN p-FETs in this work with some other reported GaN p-FETs. Our E-mode GaN p-FETs exhibit high ION/IOFF ratio and low SS.
The scaling effect on the gate length of the GaN p-FETs was also studied. Much higher |ID,max| and lower Ron are obtained (Fig. 5). By optimizing the size of the devices or directly using self-alignment structure[16, 17], the current density of GaN p-FETs will be further improved.
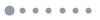
Figure 5.(Color online) (a) Output characteristics for the GaN p-FET using O3-Al2O3/HfO2 as the gate stack with LG of 1 μm. (b) Trend of |ID,max| enhancement and Ron reduction with LG scaling.
Conclusion
In this work, the leakage characteristic of O3-Al2O3 and HfO2 is investigated on the p-channel GaN device platform. The introduction of the O3-Al2O3 insertion layer can effectively reduce the leakage and increase the breakdown voltage of the O3-Al2O3/HfO2 stack. E-mode GaN p-FETs with an O3-Al2O3/HfO2 gate stack were fabricated on the p++-GaN/p-GaN/AlN/AlGaN/AlN/GaN/Si heterostructure. The fabricated GaN p-FETs possess a high current density of −4.9 mA/mm, a high ION/IOFF ratio of 6 × 106 and a low SS of 107 mV/dec, which are promising for applications in GaN CMOS logic platforms.
References
[1] K Hoo Teo, Y H Zhang, N Chowdhury et al. Emerging GaN technologies for power, RF, digital, and quantum computing applications: Recent advances and prospects. J Appl Phys, 130, 160902(2021).
[2] K F Wu, S Y Huang, W L Wang et al. Recent progress in III-nitride nanosheets: Properties, materials and applications. Semicond Sci Technol, 36, 123002(2021).
[3] E A Jones, F Wang, B Ozpineci. Application-based review of GaN HFETs., 24(2014).
[4] K J Chen, O Häberlen, A Lidow et al. GaN-on-Si power technology: Devices and applications. IEEE Trans Electron Devices, 64, 779(2017).
[5] H Amano, Y Baines, E Beam et al. The 2018 GaN power electronics roadmap. J Phys D: Appl Phys, 51, 163001(2018).
[6] W L Wang, H S Jiang, L H Li et al. Two-dimensional group-III nitrides and devices: A critical review. Rep Prog Phys, 84, 086501(2021).
[7] S J Bader, H Lee, R Chaudhuri et al. Prospects for wide bandgap and ultrawide bandgap CMOS devices. IEEE Trans Electron Devices, 67, 4010(2020).
[8] W Götz, N M Johnson, J Walker et al. Activation of acceptors in Mg-doped GaN grown by metalorganic chemical vapor deposition. Appl Phys Lett, 68, 667(1996).
[9] S J Li, P Y Sun, Z H Xing et al. Degradation mechanisms of Mg-doped GaN/AlN superlattices HEMTs under electrical stress. Appl Phys Lett, 121, 062101(2022).
[10] J O Song, J S Ha, T Y Seong. Ohmic-contact technology for GaN-based light-emitting diodes: Role of P-type contact. IEEE Trans Electron Devices, 57, 42(2010).
[11] R Chaudhuri, S J Bader, Z Chen et al. A polarization-induced 2D hole gas in undoped gallium nitride quantum wells. Science, 365, 1454(2019).
[12] T Zimmermann, M Neuburger, M Kunze et al. P-channel InGaN-HFET structure based on polarization doping. IEEE Electron Device Lett, 25, 450(2004).
[13] H Hahn, B Reuters, A Pooth et al. P-channel enhancement and depletion mode GaN-based HFETs with quaternary backbarriers. IEEE Trans Electron Devices, 60, 3005(2013).
[14] A Nakajima, Y Sumida, M H Dhyani et al. High density two-dimensional hole gas induced by negative polarization at GaN/AlGaN heterointerface. Appl Phys Express, 3, 121004(2010).
[15] N Chowdhury, J Lemettinen, Q Y Xie et al. P-channel GaN transistor based on p-GaN/AlGaN/GaN on Si. IEEE Electron Device Lett, 40, 1036(2019).
[16] N Chowdhury, Q Y Xie, J Niroula et al. Field-induced acceptor ionization in enhancement-mode GaN p-MOSFETs(2021).
[17] N Chowdhury, Q Y Xie, T Palacios. Tungsten-gated GaN/AlGaN p-FET with Imax > 120 mA/mm on GaN-on-Si. IEEE Electron Device Lett, 43, 545(2022).
[18] N Chowdhury, Q Y Xie, M Y Yuan et al. First demonstration of a self-aligned GaN p-FET(2020).
[19] Y D Yin, K B Lee. IEEE Electron Device Lett, 43, 533(2022).
[20] S J Bader, R Chaudhuri, K Nomoto et al. Gate-recessed E-mode p-channel HFET with high on-current based on GaN/AlN 2D hole gas. IEEE Electron Device Lett, 39, 1848(2018).
[21] N Chowdhury, Q Y Xie, M Y Yuan et al. Regrowth-free GaN-based complementary logic on a Si substrate. IEEE Electron Device Lett, 41, 820(2020).
[22] R M Chu, Y Cao, M Chen et al. An experimental demonstration of GaN CMOS technology. IEEE Electron Device Lett, 37, 269(2016).
[23] J Ma, G Yoo. Low subthreshold swing double-gate β-Ga2O3 field-effect transistors with polycrystalline hafnium oxide dielectrics. IEEE Electron Device Lett, 40, 1317(2019).
[24] K Mistry, C Allen, C Auth et al. A 45nm logic technology with high-k metal gate transistors, strained silicon, 9 Cu interconnect layers, 193nm dry patterning, and 100% Pb-free packaging, 247(2008).
[25] S Huang, X H Wang, X Y Liu et al. An ultrathin-barrier AlGaN/GaN heterostructure: A recess-free technology for the fabrication and integration of GaN-based power devices and power-driven circuits. Semicond Sci Technol, 36, 044002(2021).
[26] S Huang, X Y Liu, K Wei et al. O3-sourced atomic layer deposition of high quality Al2O3 gate dielectric for normally-off GaN metal-insulator-semiconductor high-electron-mobility transistors. Appl Phys Lett, 106, 033507(2015).
[27] H H Huang, G J Fang, Y Li et al. Improved and color tunable electroluminescence from n-ZnO/HfO2/p-GaN heterojunction light emitting diodes. Appl Phys Lett, 100, 233502(2012).
[28] H Jin, Q M Jiang, S Huang et al. An enhancement-mode GaN p-FET with improved breakdown voltage. IEEE Electron Device Lett, 43, 1191(2022).
[29] F Q Guo, S Huang, X H Wang et al. Appl Phys Lett, 118, 093503(2021).
[30] Z Y Zheng, W J Song, L Zhang et al. IEEE Electron Device Lett, 41, 26(2020).
[31] C Yang, H Q Fu, P Peri et al. Enhancement-mode gate-recess-free GaN-based p-channel heterojunction field-effect transistor with ultra-low subthreshold swing. IEEE Electron Device Lett, 42, 1128(2021).
[32] Z Y Zheng, W J Song, L Zhang et al. Enhancement-mode GaN p-channel MOSFETs for power integration, 525(2020).