Hooman Barati Sedeh, Natalia M. Litchinitser, "From non-scattering to super-scattering with Mie-tronics," Photonics Res. 12, 608 (2024)

Search by keywords or author
- Photonics Research
- Vol. 12, Issue 4, 608 (2024)
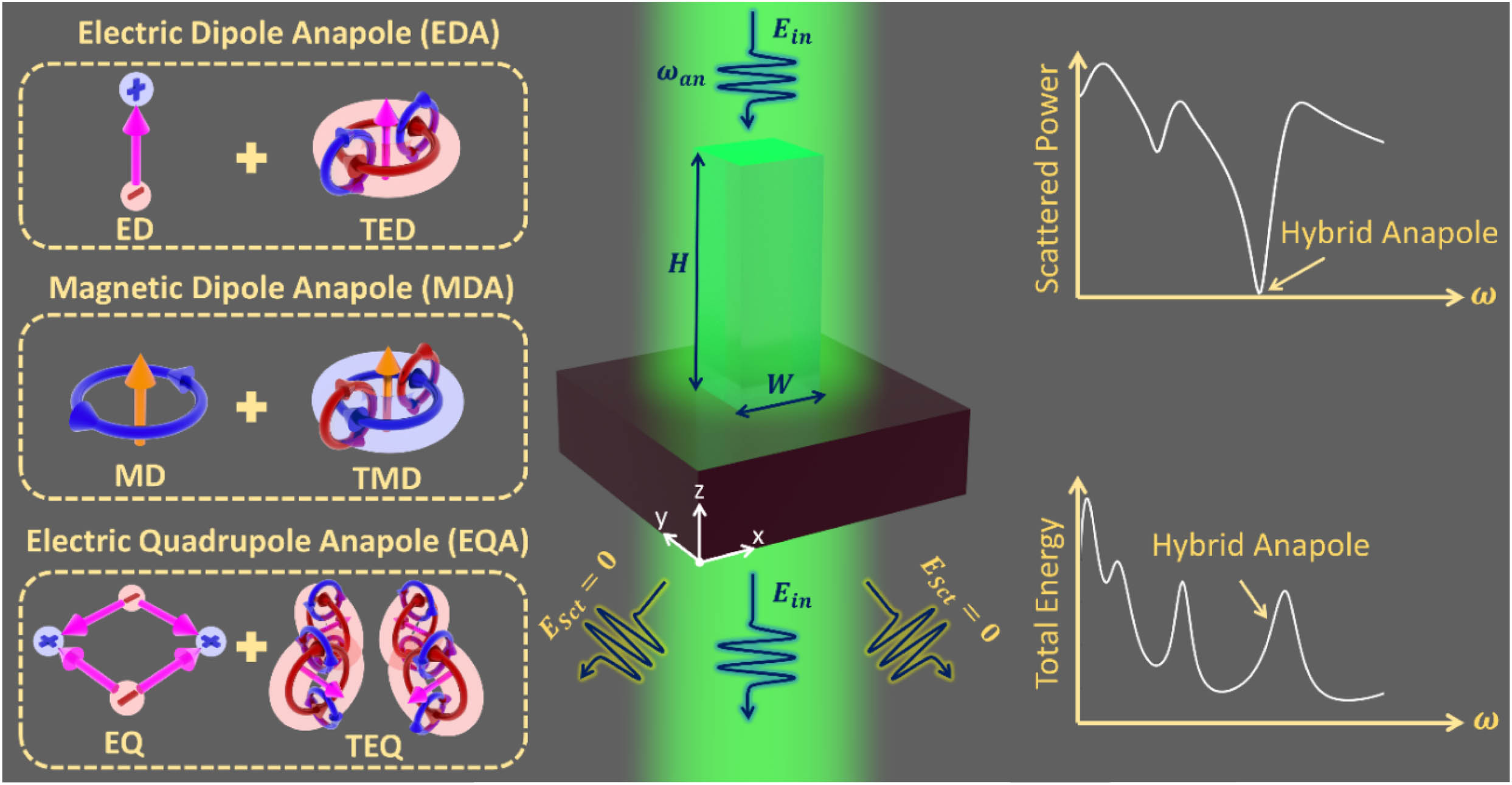
Fig. 1. Schematic representation of the formation of a hybrid anapole state within an all-dielectric cuboid meta-atom with the width and height of W = 295 nm and H = 466 nm , respectively. A monochromatic plane wave that is polarized along the x axis impinges on the meta-atom along the z axis and excites complex displacement current configurations, leading to the suppression of the far-field scattering and significant energy confinement. Inset depicts the primitive and toroidal contributions up to the electric quadrupole term with red and blue colors demonstrating the magnetic and electric field distributions, respectively.
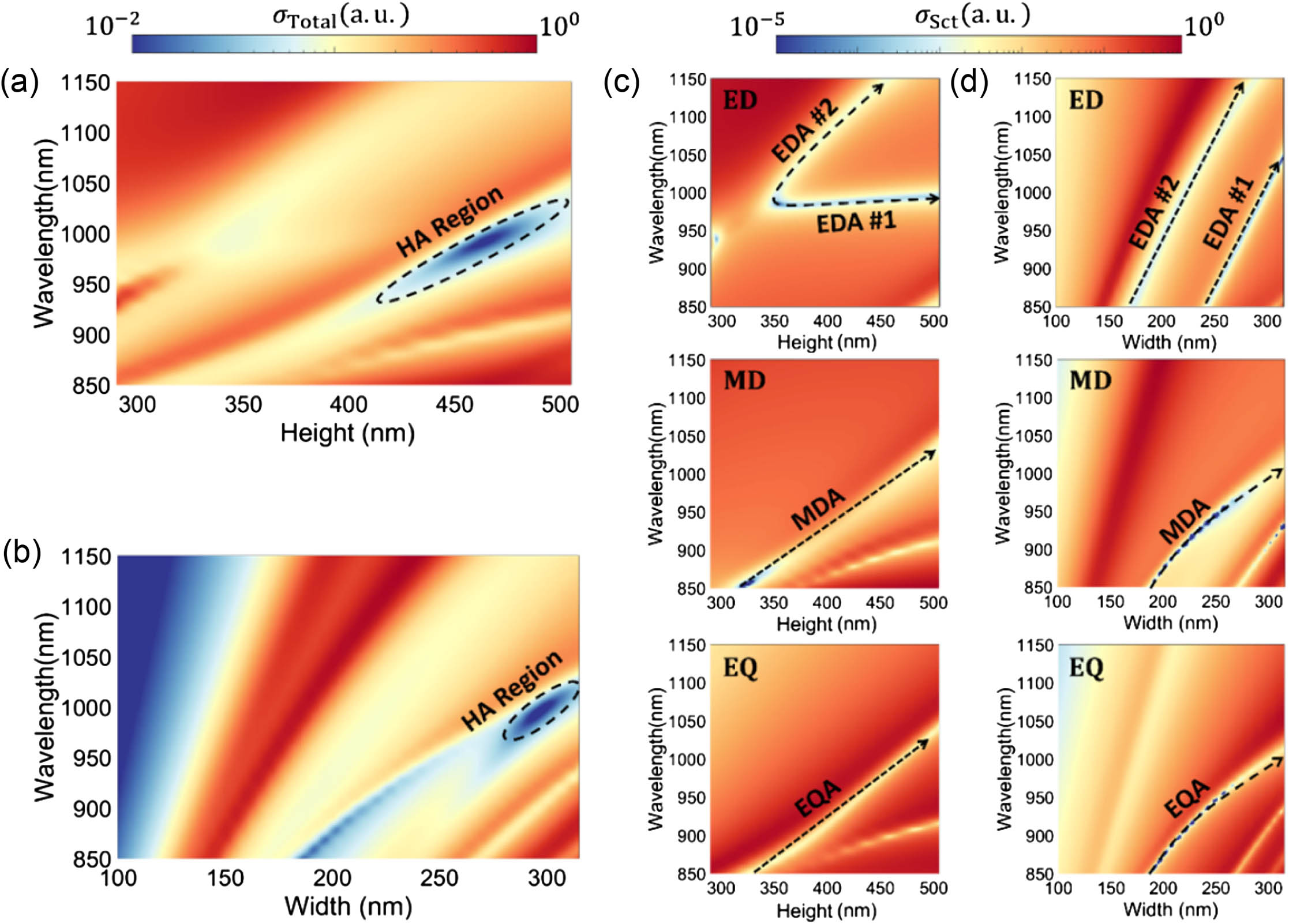
Fig. 2. Normalized total scattering cross-section of the cuboid meta-atom as a function of (a) height and (b) width and an operating wavelength under plane-wave illumination in logarithmic scale. The contribution of ED, MD, and EQ multipolar moments excited in the meta-atom as a function of (c) height and (d) width with respect to wavelength. By changing the topology of the meta-atom, several resonances emerge and disappear at certain spectral positions, leading to the formation of resonant branches that shift toward longer wavelengths as the size of the meta-atom increases.
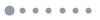
Fig. 3. Explicit contributions of amplitude and phase of primitive and toroidal moments as functions of height and wavelength for (a), (b) ED; (c), (d) MD; and (e), (f) EQ. An anapole of any kind is excited in regions wherein both conditions are simultaneously satisfied. The vertical dashed line corresponds to the dimension at which the HA state can be excited up to the EQA at the operating wavelength of λ = 990 nm (horizontal dashed line).
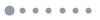
Fig. 4. Calculated amplitudes and phase differences between the primitive and toroidal contributions of a cuboid meta-atom with W = 295 nm and H = 466 nm for (a) ED, (b) MD, and (c) EQ scattering channels. In all plots, the amplitudes correspond to the left ordinate axis with a solid line representing primitive moments and dashed curves showing their toroidal counterparts, and the phase differences are read from the right ordinate axis. (d) Multipole decomposition of the cuboid meta-atom up to the magnetic quadrupole contribution. In the legend caption, “Sum” denotes the summation of the contributing moments up to the MQ term, while “Total” implies the total scattering cross-section calculated directly from COMSOL. (e) Calculated spectra corresponding to the radiated power (blue color) and stored electromagnetic energy normalized to the transparent particle (red color). The purple and magenta color bands denote the spectral positions corresponding to the dark and bright resonances, respectively, with their near-field profiles given in the insets. (f) Electric field distribution at the operating wavelength of 990 nm in three different planes of z − x , z − y , and y − x , with black arrows representing the displacement currents.
![(a) Normalized total scattering cross-section of the cuboid meta-atom under plane wave incidence with the angles θ ranging from 0° to 90° as functions of the operating wavelength. The calculated multipole decomposition results for (b) ED, (c) MD, and (d) EQ, with the dashed lines representing the regions wherein φP−φT=±π. Anapoles of any kind occur at the points where the dashed lines overlap with the scattering minima. (e) Total scattering cross-section for four different values of the incident angles θ=[0°,30°,60°,90°] as functions of wavelength. Tuning the angle of incidence results in the changes in the meta-atom’s response from non-radiative to radiative (blue line) and vice versa (red line) at two distinct wavelengths.](/Images/icon/loading.gif)
Fig. 5. (a) Normalized total scattering cross-section of the cuboid meta-atom under plane wave incidence with the angles θ ranging from 0 ° to 90 ° as functions of the operating wavelength. The calculated multipole decomposition results for (b) ED, (c) MD, and (d) EQ, with the dashed lines representing the regions wherein φ P − φ T = ± π . Anapoles of any kind occur at the points where the dashed lines overlap with the scattering minima. (e) Total scattering cross-section for four different values of the incident angles θ = [ 0 ° , 30 ° , 60 ° , 90 ° ] as functions of wavelength. Tuning the angle of incidence results in the changes in the meta-atom’s response from non-radiative to radiative (blue line) and vice versa (red line) at two distinct wavelengths.
![Schematic demonstration of achieving bianisotropic responses via breaking the inversion symmetry of the meta-atom. For (a) symmetric particles, the eigenmodes consist of either only even or odd multipoles, while for (b) asymmetric meta-atoms, the optical response consists of multipoles of mixed parities. (c) Calculated response of the pyramid meta-atom with respect to the top-to-bottom width ratio and operating wavelength. Four various points corresponding to different values of β=[0.1,0.4,0.75,1] are indicated by stars and super-scattering regime occurs once 2πσTotal3λ2>1. (d) Radiation pattern of the far-field intensity calculated at the wavelength corresponding to the super-scattering state for the meta-atoms with dimensions marked by stars in panel (c).](/Images/icon/loading.gif)
Fig. 6. Schematic demonstration of achieving bianisotropic responses via breaking the inversion symmetry of the meta-atom. For (a) symmetric particles, the eigenmodes consist of either only even or odd multipoles, while for (b) asymmetric meta-atoms, the optical response consists of multipoles of mixed parities. (c) Calculated response of the pyramid meta-atom with respect to the top-to-bottom width ratio and operating wavelength. Four various points corresponding to different values of β = [ 0.1 , 0.4 , 0.75 , 1 ] are indicated by stars and super-scattering regime occurs once 2 π σ Total 3 λ 2 > 1 . (d) Radiation pattern of the far-field intensity calculated at the wavelength corresponding to the super-scattering state for the meta-atoms with dimensions marked by stars in panel (c).
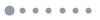
Fig. 7. Calculated amplitudes and phase differences between the electric primitive and first- and second-type toroidal multipoles of a cuboid meta-atom with W = 295 nm and H = 466 nm . The amplitudes correspond to the left ordinate axis with a solid line representing the primitive moment, a dashed curve denoting the first type of electric toroidal moment, and a circle graph showing the second type of toroidal moment, and the phase differences are read from the right ordinate axis.
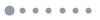
Fig. 8. Real (blue line) and imaginary (red curve) parts of polysilicon refractive index.
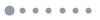
Fig. 9. Contribution of MQ moment excited within the cuboid scatterer as a function of (a) height and (b) width with respect to wavelength. Changing the height of the meta-atom has a negligible effect on the MQ response, while the variation of its width can significantly shift the scattering response. (c), (d) Contributions of both primitive and toroidal MQ multipole moments as functions of height and wavelength. At the spectral position wherein the optical response of the meta-atom is mainly governed by higher-order anapole states up to EQA (shown with black dashed lines), the conditions for satisfying magnetic quadrupole anapole are not fulfilled.
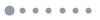
Fig. 10. Explicit dynamics of (a) amplitude and (b) phase of primitive and toroidal contributions as functions of height and wavelength for ED, MD, EQ, and MQ. An anapole of any kind is excited in regions wherein both conditions are simultaneously satisfied. The vertical dashed line corresponds to the dimension at which the HA state can be excited up to the EQA at the operating wavelength of λ = 990 nm (horizontal dashed line).
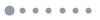
Fig. 11. (a) Evolution of the scattering cross-section under the continuous change of the diameter-to-height aspect ratio. (b) Multipole decomposition and (c) stored energy spectra for a cylindrical particle with D = 605 nm and H = 50 nm . (d) Optical response of the cylindrical meta-atom as a function of diameter and wavelength. By changing the diameter, the spectral position of the desired electric dipole anapole can be tuned. (e) The near-field distribution of first-, second-, and third-order electric anapole states at their corresponding excited wavelengths.
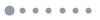
Fig. 12. Calculated total scattering cross-section (blue color) and stored energy (red color) of two meta-atoms supporting HA (solid line) and first-order EDA (dashed curve) as a function of the operating wavelength. The scattered field’s x and z components at the corresponding wavelength of anapole excitation are also given as a point of comparison.
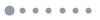
Fig. 13. Calculated scattering cross-sections of the cuboid meta-atom for (a) ED, (b) MD, (c) EQ, and (d) MQ multipole moments as functions of β and the operating wavelength. The asymmetric parameter corresponding to the inversion symmetry can be used as another degree of freedom to manipulate the emerged resonant dips within the scattering spectra.
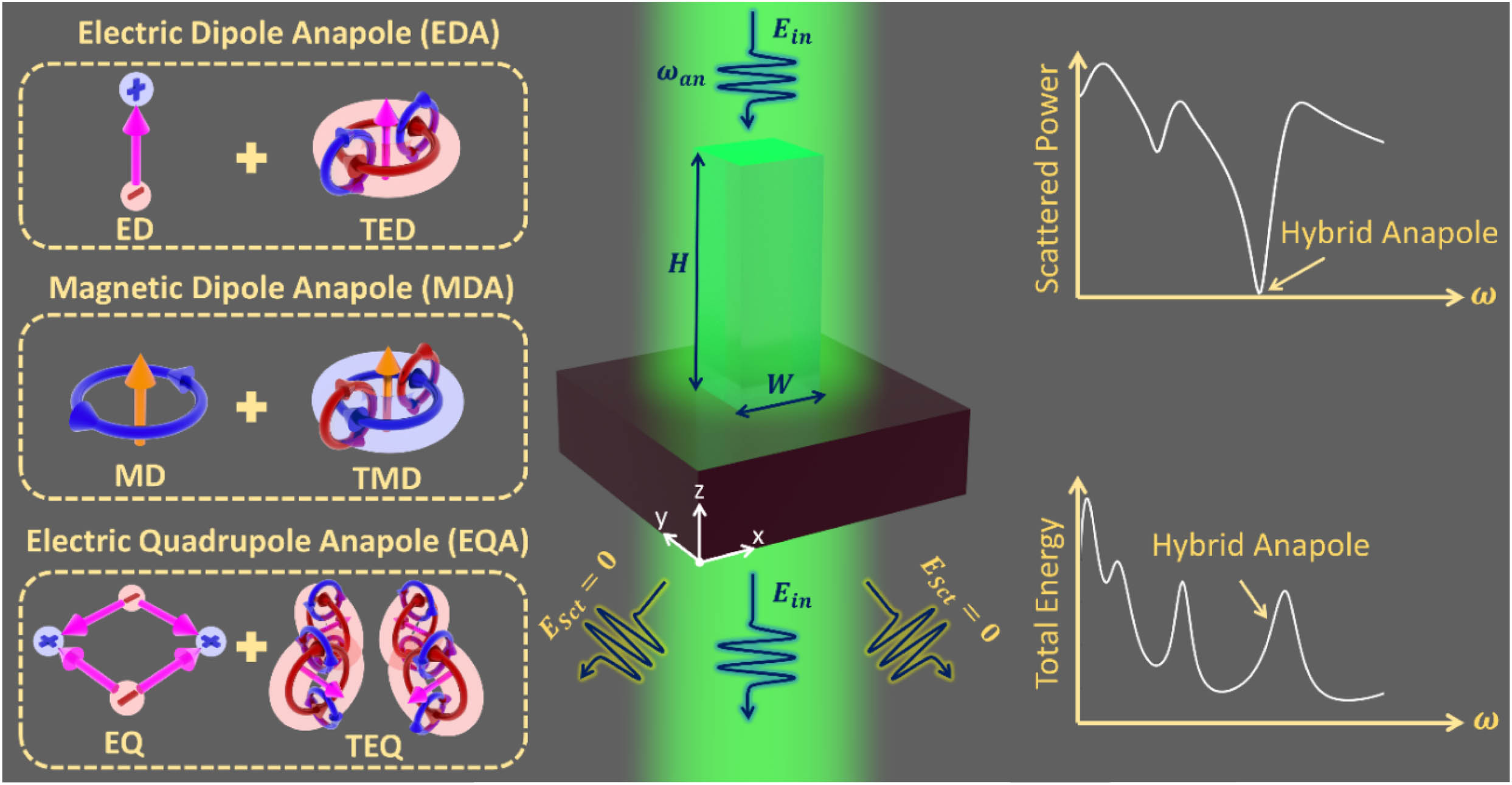
Set citation alerts for the article
Please enter your email address