Jinheng Du, Wei Song, Huaijin Zhang. Advances in Three-Dimensional Quasi-Phase Matching[J]. Chinese Journal of Lasers, 2021, 48(12): 1208001

Search by keywords or author
- Chinese Journal of Lasers
- Vol. 48, Issue 12, 1208001 (2021)
![Principle diagram of birefringence phase matching based on negative uniaxial crystal[21]](/richHtml/zgjg/2021/48/12/1208001/img_1.jpg)
Fig. 1. Principle diagram of birefringence phase matching based on negative uniaxial crystal[21]
![Schematic of relationship between frequency doubling laser intensity and propagation distance under different phase matching cases[31]. (a) Full phase matching; (b) first-order quasi-phase matching; (c) phase mismatching](/richHtml/zgjg/2021/48/12/1208001/img_2.jpg)
Fig. 2. Schematic of relationship between frequency doubling laser intensity and propagation distance under different phase matching cases[31]. (a) Full phase matching; (b) first-order quasi-phase matching; (c) phase mismatching
![Schematic of nonlinear coefficient distribution for one-dimensional quasi-phase matching[31]](/Images/icon/loading.gif)
Fig. 3. Schematic of nonlinear coefficient distribution for one-dimensional quasi-phase matching[31]
![Structural diagram of three-dimensional nonlinear optical crystal with simple cubic lattice[21]](/Images/icon/loading.gif)
Fig. 4. Structural diagram of three-dimensional nonlinear optical crystal with simple cubic lattice[21]
![QPM mechanism in laser-engineered LN crystal[48]. (a) Comparison among full phase matching,QPM based on poled LN crystal, QPM based on laser-engineered LN crystal and phase mismatching; (b) structural diagram of 3D NPC fabricated by femtosecond laser direct writing](/Images/icon/loading.gif)
Fig. 5. QPM mechanism in laser-engineered LN crystal[48]. (a) Comparison among full phase matching,QPM based on poled LN crystal, QPM based on laser-engineered LN crystal and phase mismatching; (b) structural diagram of 3D NPC fabricated by femtosecond laser direct writing
![Sample characterization. (a) Image of 3D structure recorded using C˙erenkov-type second harmonic confocal microscopy[61]; (b)second harmonic image in x-y plane taken through confocal second harmonic microscopic system[62]; (c) intensity distribution along central black line in Fig.6(b)[48]](/Images/icon/loading.gif)
Fig. 6. Sample characterization. (a) Image of 3D structure recorded using erenkov-type second harmonic confocal microscopy[61]; (b)second harmonic image in x-y plane taken through confocal second harmonic microscopic system[62]; (c) intensity distribution along central black line in Fig.6 (b)[48]
![Demonstration of second harmonic generation process in 3D LN NPC[48]. (a) 3D reciprocal lattice array and typical 3D reciprocal vectors; (b)-(e) measured and simulated 3D QPM second harmonic beam patterns for various input fundamental wavelengths with QPM modulation and corresponding reciprocal vectors shown in right column](/Images/icon/loading.gif)
Fig. 7. Demonstration of second harmonic generation process in 3D LN NPC[48]. (a) 3D reciprocal lattice array and typical 3D reciprocal vectors; (b)-(e) measured and simulated 3D QPM second harmonic beam patterns for various input fundamental wavelengths with QPM modulation and corresponding reciprocal vectors shown in right column
![Dependence of second harmonic power on input parameters[48]. (a) Dependence of second harmonic power on fundamental wavelength; (b) relationship between QPM second harmonic power and input pump power at fundamental wavelength of 829 nm](/Images/icon/loading.gif)
Fig. 8. Dependence of second harmonic power on input parameters[48]. (a) Dependence of second harmonic power on fundamental wavelength; (b) relationship between QPM second harmonic power and input pump power at fundamental wavelength of 829 nm
![Principle diagram of QPM waveguide[63]. (a) Design diagram of QPM waveguide; schemes to modulate χ(2)nonlinearity inside QPM waveguide core: (b) one period (A), (c) two periods (A, B), and (d) four periods (A, B, C, D)](/Images/icon/loading.gif)
Fig. 9. Principle diagram of QPM waveguide[63]. (a) Design diagram of QPM waveguide; schemes to modulate χ(2)nonlinearity inside QPM waveguide core: (b) one period (A), (c) two periods (A, B), and (d) four periods (A, B, C, D)
![Sample characterization[21]. (a) SEM image of domain structure of c-direction BCT crystal after corrosion; (b) SEM image of domain structure of a-direction BCT crystal after corrosion; (c) statistical width distribution of random domains in BCT crystal; (d) structural diagram of random 3D stacking domain in BCT crystal](/Images/icon/loading.gif)
Fig. 10. Sample characterization[21]. (a) SEM image of domain structure of c-direction BCT crystal after corrosion; (b) SEM image of domain structure of a-direction BCT crystal after corrosion; (c) statistical width distribution of random domains in BCT crystal; (d) structural diagram of random 3D stacking domain in BCT crystal
![3D QPM process with random domain modulation in self-polarized BCT crystal[21]](/Images/icon/loading.gif)
Fig. 11. 3D QPM process with random domain modulation in self-polarized BCT crystal[21]
![Schematic of experimental setup for 3D QPM frequency doubling using BCT crystal[21]](/Images/icon/loading.gif)
Fig. 12. Schematic of experimental setup for 3D QPM frequency doubling using BCT crystal[21]
![Frequency-doubled laser spots obtained (left) by 3D QPM frequency doubling experiment using BCT crystal and (right) by theoretical simulation [21]](/Images/icon/loading.gif)
Fig. 13. Frequency-doubled laser spots obtained (left) by 3D QPM frequency doubling experiment using BCT crystal and (right) by theoretical simulation [21]
![Frequency-doubled laser power versus fundamental power with spectral curves of 1100 nm fundamental laser and its frequency-doubled laser shown in insets (1) and (2), respectively[21]](/Images/icon/loading.gif)
Fig. 14. Frequency-doubled laser power versus fundamental power with spectral curves of 1100 nm fundamental laser and its frequency-doubled laser shown in insets (1) and (2), respectively[21]
![Schematic of experimental setup for femtosecond laser direct writing of 3D ferroelectric domain structure[67]](/Images/icon/loading.gif)
Fig. 15. Schematic of experimental setup for femtosecond laser direct writing of 3D ferroelectric domain structure[67]
![NPC and corresponding inverted lattice vectors[67]. (a) Two-dimensional NPC diagram; (b) 3D NPC diagram; (c) Čerenkov second harmonic micrograph of 3D ferroelectric domain pattern fabricated in BCT crystal by femtosecond laser pulse](/Images/icon/loading.gif)
Fig. 16. NPC and corresponding inverted lattice vectors[67]. (a) Two-dimensional NPC diagram; (b) 3D NPC diagram; (c) Čerenkov second harmonic micrograph of 3D ferroelectric domain pattern fabricated in BCT crystal by femtosecond laser pulse
![Ferroelectric domain reversal in BCT crystal realized by using femtosecond laser[67]. (a) Laser direct writing of ferroelectric domain structure in y-z plane with θ-like domain structure observed by using Čherenkov second harmonic microscope, in which background (weak random patterns around θ domain) originates from random submicron periodic domain structure inherent in BCT crystal; mechanism of formation of θ-shaped domain wall structure in BCT crystals: (b) temperature gradient induced by nonlinear absorption of near-infrared laser by BCT crystal (arrows pointing to high temperature region); (c) projection of temperature gradient along z-axis direction at different positions around focal point; (d) pair of oppositely oriented thermoelectric fields used to polarize (e) originally disordered random domains into (f) pair of head-to-head ferroelectric domains](/Images/icon/loading.gif)
Fig. 17. Ferroelectric domain reversal in BCT crystal realized by using femtosecond laser[67]. (a) Laser direct writing of ferroelectric domain structure in y-z plane with θ-like domain structure observed by using Čherenkov second harmonic microscope, in which background (weak random patterns around θ domain) originates from random submicron periodic domain structure inherent in BCT crystal; mechanism of formation of θ-shaped domain wall structure in BCT crystals: (b) temperature gradient induced by nonlinear absorption of near-infrared laser by BCT crystal (arrows pointing to high temperature region); (c) projection of temperature gradient along z-axis direction at different positions around focal point; (d) pair of oppositely oriented thermoelectric fields used to polarize (e) originally disordered random domains into (f) pair of head-to-head ferroelectric domains
![3D QPM frequency-doubling phenomenon[67]. (a) Far-field frequency-doubling spot pattern observed in simple tetragonal ferroelectric domain structure with frequency-doubling light wavelength of 800 nm; (b) schematic of 3D QPM of 3D ferroelectric domain structure modulation; (c) divergence outer angle of frequency-doubling loop with h=-1 versus fundamental wavelength and Λx(curve represents theoretical value, hollow point represents measured value); (d) experimental and (e) theoretical distributions of frequency-doubling bright spots on Čherenkov ring with h=-2; (f) relationship between fundamental frequency optical power and colinear frequency-doubling optical power modulated by 3D domain structure with Λx=25.6 μm (hollow point represents measured value, and solid curve represents quadratic fitting curve), and inset shows frequency doubling optical power as a function of wavelength after annealing (solid point represents experimental value, and curve represents theoretical calculation value)](/Images/icon/loading.gif)
Fig. 18. 3D QPM frequency-doubling phenomenon[67]. (a) Far-field frequency-doubling spot pattern observed in simple tetragonal ferroelectric domain structure with frequency-doubling light wavelength of 800 nm; (b) schematic of 3D QPM of 3D ferroelectric domain structure modulation; (c) divergence outer angle of frequency-doubling loop with h=-1 versus fundamental wavelength and Λx(curve represents theoretical value, hollow point represents measured value); (d) experimental and (e) theoretical distributions of frequency-doubling bright spots on Čherenkov ring with h=-2; (f) relationship between fundamental frequency optical power and colinear frequency-doubling optical power modulated by 3D domain structure with Λx=25.6 μm (hollow point represents measured value, and solid curve represents quadratic fitting curve), and inset shows frequency doubling optical power as a function of wavelength after annealing (solid point represents experimental value, and curve represents theoretical calculation value)
![Erasable properties of domain structures in BCT crystals[67]. (a) Čherenkov second harmonic microscopic image of BCT after annealing below Curie point; (b) Čherenkov second harmonic microscopic image of BCT after annealing above Curie point](/Images/icon/loading.gif)
Fig. 19. Erasable properties of domain structures in BCT crystals[67]. (a) Čherenkov second harmonic microscopic image of BCT after annealing below Curie point; (b) Čherenkov second harmonic microscopic image of BCT after annealing above Curie point
![Sample characterization[75]. (a) Phase diagram of KTN crystal structure; (b) photoelectron peaks of Ta 4f and Nb 3d in KTN crystal and their corresponding fitting curves; (c) electrical hysteresis loop of KTN crystal; (d) endothermic and exothermic effect curves of KTN crystal measured by differential scanning calorimetry (DSC)](/Images/icon/loading.gif)
Fig. 20. Sample characterization[75]. (a) Phase diagram of KTN crystal structure; (b) photoelectron peaks of Ta 4f and Nb 3d in KTN crystal and their corresponding fitting curves; (c) electrical hysteresis loop of KTN crystal; (d) endothermic and exothermic effect curves of KTN crystal measured by differential scanning calorimetry (DSC)
![Sample characterization[75]. (a) Image of ferroelectric domain structure on a-b plane of KTN crystal under polarized light microscope; (b) statistical distribution result of period length for KTN crystal 180° random domain](/Images/icon/loading.gif)
Fig. 21. Sample characterization[75]. (a) Image of ferroelectric domain structure on a-b plane of KTN crystal under polarized light microscope; (b) statistical distribution result of period length for KTN crystal 180° random domain
![Crystal structure and ferroelectric domain distributions of KTN[75]. (a) Schematic of cubic-like domain of natural Rubik's cube structure in KTN crystal; (b) structure model of x-y plane ferroelectric domain (out-of-plane) of KTN crystal with × and · indicating internal and external polarization; (c) structure model of x-y plane ferroelectric domain (in-plane) of KTN crystal; (d) vertical PFM amplitude image and (e) transverse PFM phase image of KTN x-y plane in same area with scale bar of 2 μm](/Images/icon/loading.gif)
Fig. 22. Crystal structure and ferroelectric domain distributions of KTN[75]. (a) Schematic of cubic-like domain of natural Rubik's cube structure in KTN crystal; (b) structure model of x-y plane ferroelectric domain (out-of-plane) of KTN crystal with × and · indicating internal and external polarization; (c) structure model of x-y plane ferroelectric domain (in-plane) of KTN crystal; (d) vertical PFM amplitude image and (e) transverse PFM phase image of KTN x-y plane in same area with scale bar of 2 μm
![Linear optical response of KTN crystal[75]. (a) Bragg diffraction diagram of KTN supercell; visible laser Bragg diffraction spots of (b) a cut and (c) c cut KTN crystal samples at room temperature](/Images/icon/loading.gif)
Fig. 23. Linear optical response of KTN crystal[75]. (a) Bragg diffraction diagram of KTN supercell; visible laser Bragg diffraction spots of (b) a cut and (c) c cut KTN crystal samples at room temperature
![Nonlinear optical response of KTN crystal[75]. (a) Schematic of near-field second harmonic spot imaging of KTN supercell; second harmonic microscopy images of (b) b-c and (c) a-b planes in KTN crystal with scale bar of 25 μm](/Images/icon/loading.gif)
Fig. 24. Nonlinear optical response of KTN crystal[75]. (a) Schematic of near-field second harmonic spot imaging of KTN supercell; second harmonic microscopy images of (b) b-c and (c) a-b planes in KTN crystal with scale bar of 25 μm
![Statistical distributions of supercells[75]. (a)(b) Statistical distributions of supercells in x-direction KTN crystal; (c)(d) statistical distributions of supercells in z-direction KTN crystal](/Images/icon/loading.gif)
Fig. 25. Statistical distributions of supercells[75]. (a)(b) Statistical distributions of supercells in x-direction KTN crystal; (c)(d) statistical distributions of supercells in z-direction KTN crystal
![Second harmonic generation based on 3D KTN crystal generated by experiment and simulation[75]. (a) Schematic of 3D inverse lattice vector of KTN crystal supercell; theoretical fitting of each QPM process in KTN supercell and comparison with experimental results: (b) two-dimensional nonlinear Bragg diffraction spot; (c) second harmonic spot generated by collinear QPM; (d)(e) second harmonic spots generated by interaction of fundamental frequency diffracted laser with two-dimensional inverse lattice vector in vertical plane of incident laser; (f)-(h) second harmonic spots produced by interaction of fundamental frequency diffracted laser with inverse lattice vector in direction of incident laser; (i) comparison of second harmonic results obtained by experiment and theoretical fitting](/Images/icon/loading.gif)
Fig. 26. Second harmonic generation based on 3D KTN crystal generated by experiment and simulation[75]. (a) Schematic of 3D inverse lattice vector of KTN crystal supercell; theoretical fitting of each QPM process in KTN supercell and comparison with experimental results: (b) two-dimensional nonlinear Bragg diffraction spot; (c) second harmonic spot generated by collinear QPM; (d)(e) second harmonic spots generated by interaction of fundamental frequency diffracted laser with two-dimensional inverse lattice vector in vertical plane of incident laser; (f)-(h) second harmonic spots produced by interaction of fundamental frequency diffracted laser with inverse lattice vector in direction of incident laser; (i) comparison of second harmonic results obtained by experiment and theoretical fitting
![Second harmonic intensity distribution images for different polarizations[75]. (a) Schematic of experimental setup for 3D QPM second harmonic generation; (b) second harmonic spot distribution when incident fundamental laser is polarized along b-axis; (c) second harmonic spot distribution when incident fundamental laser is polarized along c-axis; (d) relative intensity of second harmonic laser at different polarization states when incident fundamental laser is polarized along b-axis; (e) relative intensity of second harmonic laser at different polarization states when incident fundamental laser is polarized along c-axis; (f) quadratic relationship curve between second harmonic laser power and fundamental laser power](/Images/icon/loading.gif)
Fig. 27. Second harmonic intensity distribution images for different polarizations[75]. (a) Schematic of experimental setup for 3D QPM second harmonic generation; (b) second harmonic spot distribution when incident fundamental laser is polarized along b-axis; (c) second harmonic spot distribution when incident fundamental laser is polarized along c-axis; (d) relative intensity of second harmonic laser at different polarization states when incident fundamental laser is polarized along b-axis; (e) relative intensity of second harmonic laser at different polarization states when incident fundamental laser is polarized along c-axis; (f) quadratic relationship curve between second harmonic laser power and fundamental laser power
![Experimental results[75]. (a) Broadband second harmonic spectra of a-direction KTN crystal; second harmonic power versus incident power for fundamental laser wavelengths of (b) 900 nm, (c) 1040 nm, and (d)1160 nm](/Images/icon/loading.gif)
Fig. 28. Experimental results[75]. (a) Broadband second harmonic spectra of a-direction KTN crystal; second harmonic power versus incident power for fundamental laser wavelengths of (b) 900 nm, (c) 1040 nm, and (d)1160 nm
|
Table 1. Common ferroelectric crystals and their basic ferroelectric properties[31]
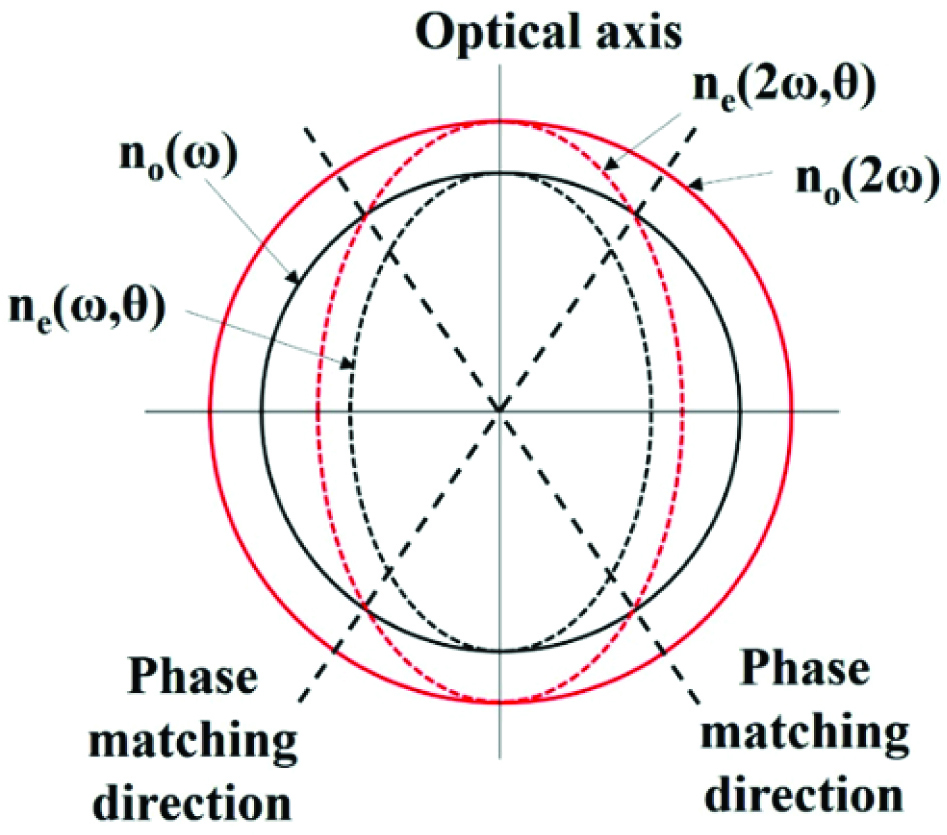
Set citation alerts for the article
Please enter your email address