Dongyi Shen, Guolin Zhao, Xianfeng Chen, Wenjie Wan, "Smith–Purcell radiation-like photoacoustic phased array," Chin. Opt. Lett. 21, 041901 (2023)

Search by keywords or author
- Chinese Optics Letters
- Vol. 21, Issue 4, 041901 (2023)
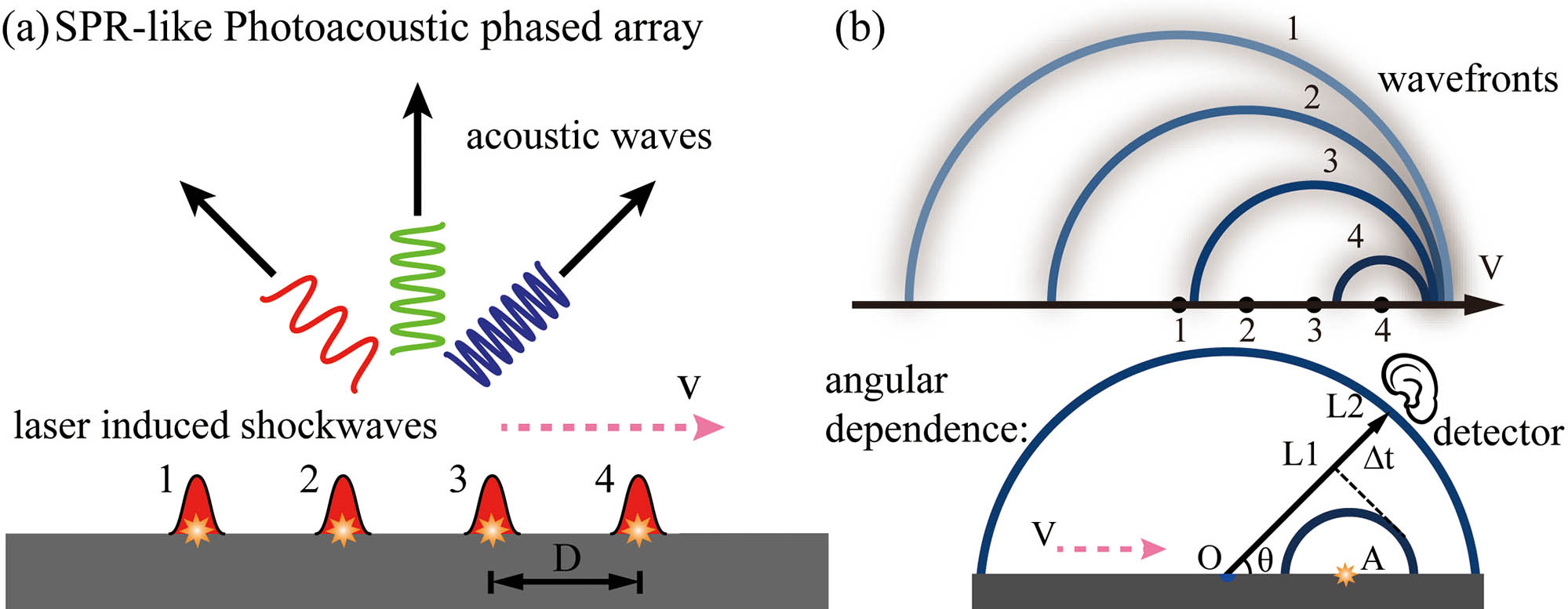
Fig. 1. Schematic of SPR-like photoacoustic phased array in a degraded form. (a) SPR-like photoacoustic phased array in a degraded form; the sequentially (1→4) excited laser-shock array emits acoustic radiation into the far field. (b) Upper, the resultant multiple propagating wavefronts from the procedure in (a); integers 1→4 denote each source and every wavefront in time sequence (indicated by the color). Lower, the effective quasi-phase line (dashed, normal to OL1 and OL2) depicts the angle dependence in the broadband SPR-like pattern in (b). By the moment when the detector captures the first shock front (blue) from source O, the secondary shock front from source A propagates to the detector. The delay time (Δt) for the detector to catch the later shock wave varies with the detection angle; accordingly, the received frequency is also changed.
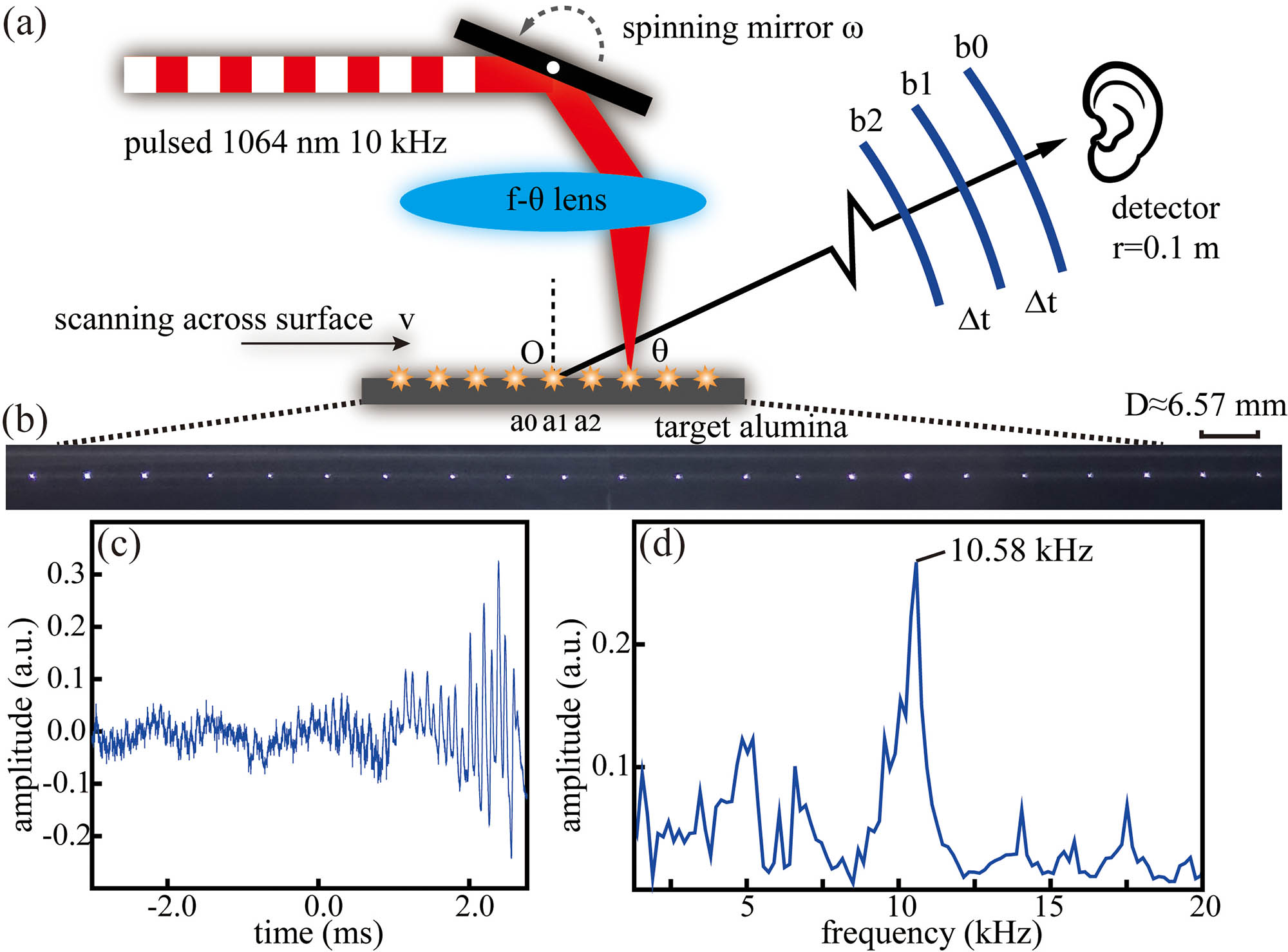
Fig. 2. Experimental observation of SPR-like photoacoustic radiation from a linear phased array in the far field. (a) Given a rotating mirror with a stable angular speed ω, the pump is scanned and focused onto the target alumina plate sequentially in the order a0→a1→a2, forming an effective laser-induced plasma shock wave phased array. The detector is placed r = 0.1 m from the array center O. The detector acquires the SPR signals also in the order b0→b1→b2. For a fixed detection angle θ and rotating ω, the time delay Δt remains unchanged and irrelevant to the detector’s distance in the far field. (b) Snapshot of such an SPR-like linear phased array by laser-induced plasma; the periodicity D is about 6.57 mm. (c) Measured real-time temporal signal and (d) its corresponding spectrum with a peak frequency at 10.58 kHz. The signal is collected and processed at a surface scanning speed of 10 m/s (effective periodicity ∼1 mm) at θ = 0°.
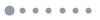
Fig. 3. Angular and velocity dependence of the phased array emission in the far field. (a) and (b) testify to the velocity-dependent frequency with fixed detection angles 0° and 30°, respectively. Insets in (a) and (b) are the spectrum collected under the condition with surface velocity 65.7 m/s (effective grating period 6.57 mm) and 140.1 m/s (effective grating period 14.01 mm). (c) and (d) demonstrate the angular dependence in the photoacoustic SPR specially picked at surface velocities of 65.7 m/s and 140.1 m/s. The dots are experimental measurements, and the solid lines are the theoretical curves calculated by Eq. (1 ).
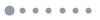
Fig. 4. Experimental (solid blue) and theoretical (black) radiation spectra at (a) θ = 0° and (b) θ = 30°; (a) N = 25 (number of acoustic sources on the target), vs = 65.7 m/s, θ = 0°; (b) N = 11, vs = 140.1 m/s, θ = 30°. The directly detected temporal signals are averaged 128 times before fast Fourier transform (FFT) processing into experimental data via an oscilloscope. Note that the dashed black curves (r = 0.1 m) are calculated by directly summing up all the phase-delayed acoustic sources and gathering radiated acoustic pressure amplitude at given conditions. The detection angle θ for r = 0.1 m calculation is defined from the center of the array.
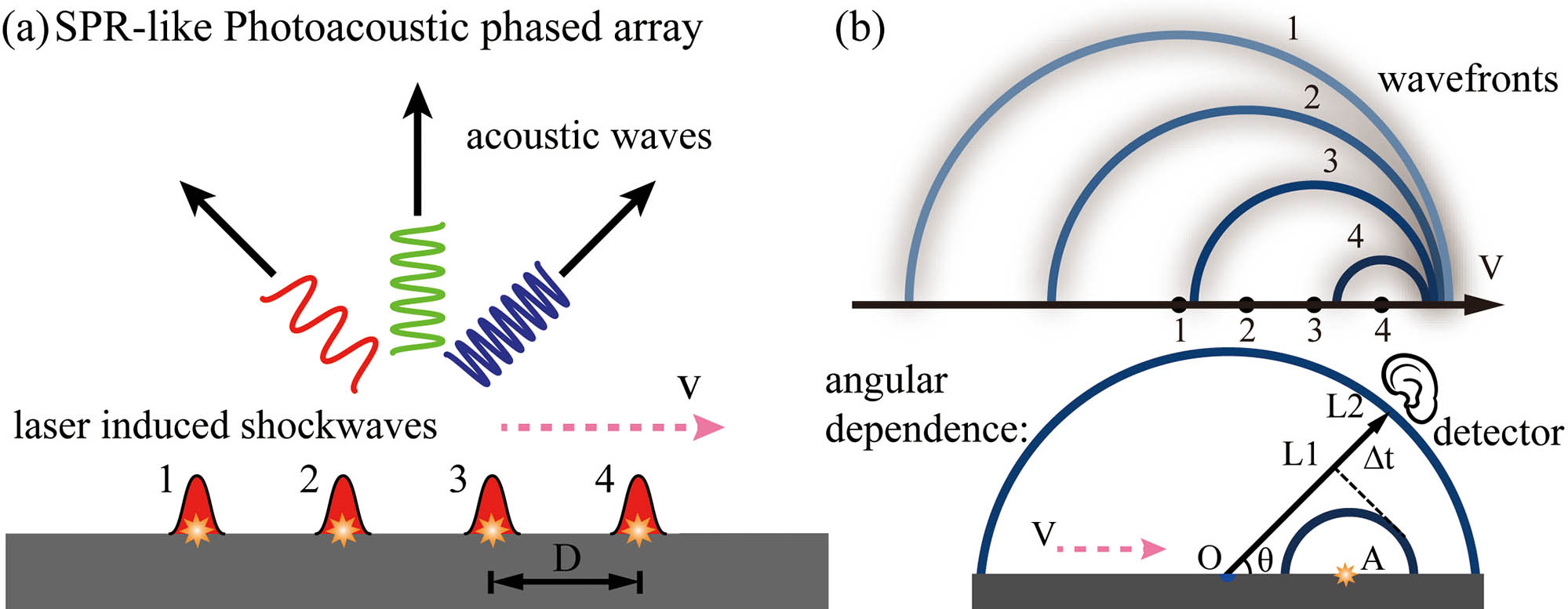
Set citation alerts for the article
Please enter your email address