Shifei Zhang, Lingling Huang, Guangzhou Geng, Junjie Li, Xiaowei Li, Yongtian Wang, "Full-Stokes polarization transformations and time sequence metasurface holographic display," Photonics Res. 10, 1031 (2022)

Search by keywords or author
- Photonics Research
- Vol. 10, Issue 4, 1031 (2022)
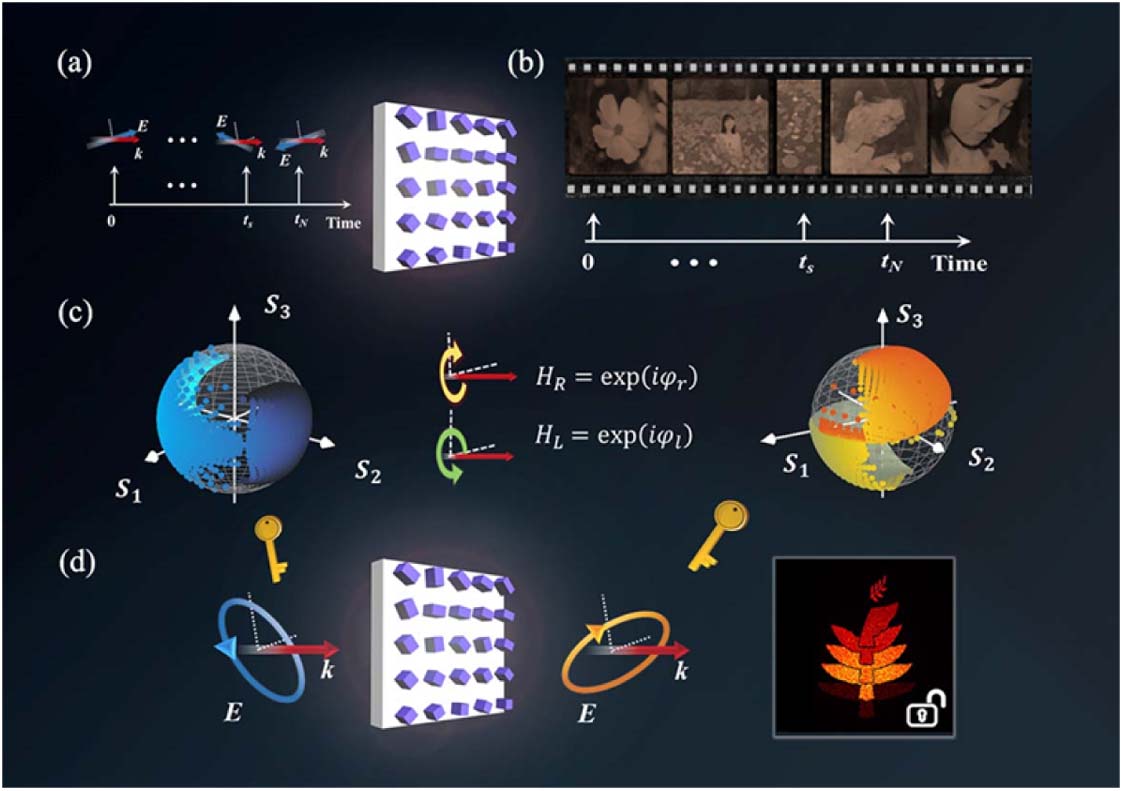
Fig. 1. Principle of polarization multiplexed holography for time sequence holographic video and optical encryption based on metasurfaces. (a) Time-dependent image frames encoded in diverse linear polarization states ranging from zero to π . (b) Holographic video display addressed by varied input and fixed output polarizations. (c) Flexible mapping from the input Poincaré sphere to the output Poincaré sphere, available by designing the phase-only holograms of RCP and LCP channels. (d) Optical encryption that uses input and output polarization states as double secret keys.
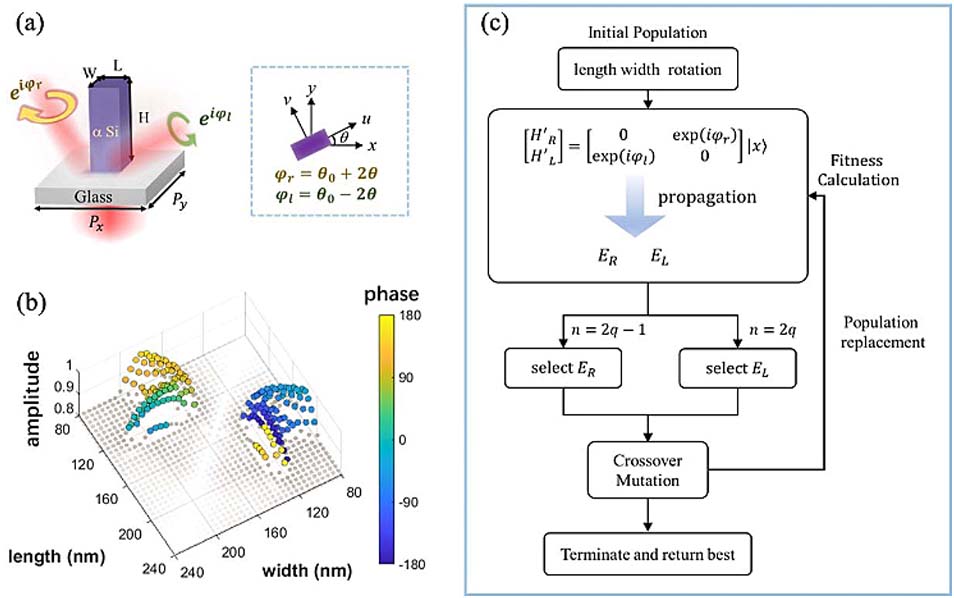
Fig. 2. Metasurface design and digitalization based on hybrid genetic algorithm. (a) Schematic illustration of phase modulation by combining dynamic phase and geometric phase. (b) Simulated results for the amplitude and dynamic phase of t r l channel. The selected nanofins are marked by colored dots, with various phases ranging from − π to π and high amplitude above 0.83. (c) Flowchart of the hybrid genetic algorithm for generating metasurfaces based on the selected structures, where n is an integer that represents the parity of iteration number.
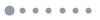
Fig. 3. Scanning electron microscopy images of the fabricated samples and the experimental setup. (a), (b) Scanning electron microscopy images of sample 1 (time sequence holographic video) and sample 2 (optical encryption), composed of diverse cross sections and rotations. (c) Experimental setup for vectorial holography, which works at various input/output polarization channels. P 1 and P 2 , polarizers; HWP, half-wave plate; QWP, quarter-wave plate; L 1 and L 2 , lenses; OBJ, objective.
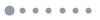
Fig. 4. Design principle of the time sequence holographic video of Tagore’s poem. (a) Time-dependent frames encoded in the linearly polarized input, which round the equator of the Poincaré sphere. (b) The preset phase modulation φ j of the j th word covers 0°–180°, and 16 vectorial words would be generated in one polarization modulation period. (c) Simulated and experimental results for dynamic display; blue polarization states represent the input, and orange states represent the output (see Visualization 1 ).
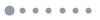
Fig. 5. Design principle of the optical encryption with double secret keys. (a) First secret key provided by input polarization states. The blue dots represent some deceptive keys, and the blue star marked at x -polarization is the correct key. (b) Polarization encryption rules encoded by φ j and χ j that change the phase delay and amplitude difference of vectorial images. (c) Encrypted images with only x -polarized input (first secret key). (d) Second secret key provided by output polarization states. Among those possibilities, the orange star that represents y -polarized output is the second correct key. (e) Simulated and experimental results for encrypted images at diverse input/output polarization channels (see Visualization 2 ).
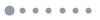
Fig. 6. Experimental results of sample 1 at diverse input and output polarization selections. The blue arrows and orange arrows are polarization states of input and output, respectively.
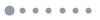
Fig. 7. Experimental results of sample 2 at six diverse input and output polarization selections. The blue arrows and orange arrows are polarization states of input and output, respectively.
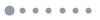
Fig. 8. Theoretical results of sample 2 at six diverse input and output polarization selections. The blue arrows and orange arrows are polarization states of input and output, respectively.
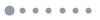
Fig. 9. Conversion efficiency and broadband behavior of sample 1. (a) Data processing of holographic construction efficiency, using ROIs generated by the algorithm to extract effective information. (b) Polarization conversion efficiencies of four circularly polarized channels. (c) Conversion efficiencies η r l and η l r of polarization multiplexed holography.
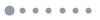
Fig. 10. Conversion efficiency and broadband behavior of sample 2. (a) Data processing of holographic construction efficiency, using ROIs generated by the algorithm to extract effective information. (b) Polarization conversion efficiencies of four circularly polarized channels. (c) Conversion efficiencies η r l and η l r of polarization multiplexed holography.
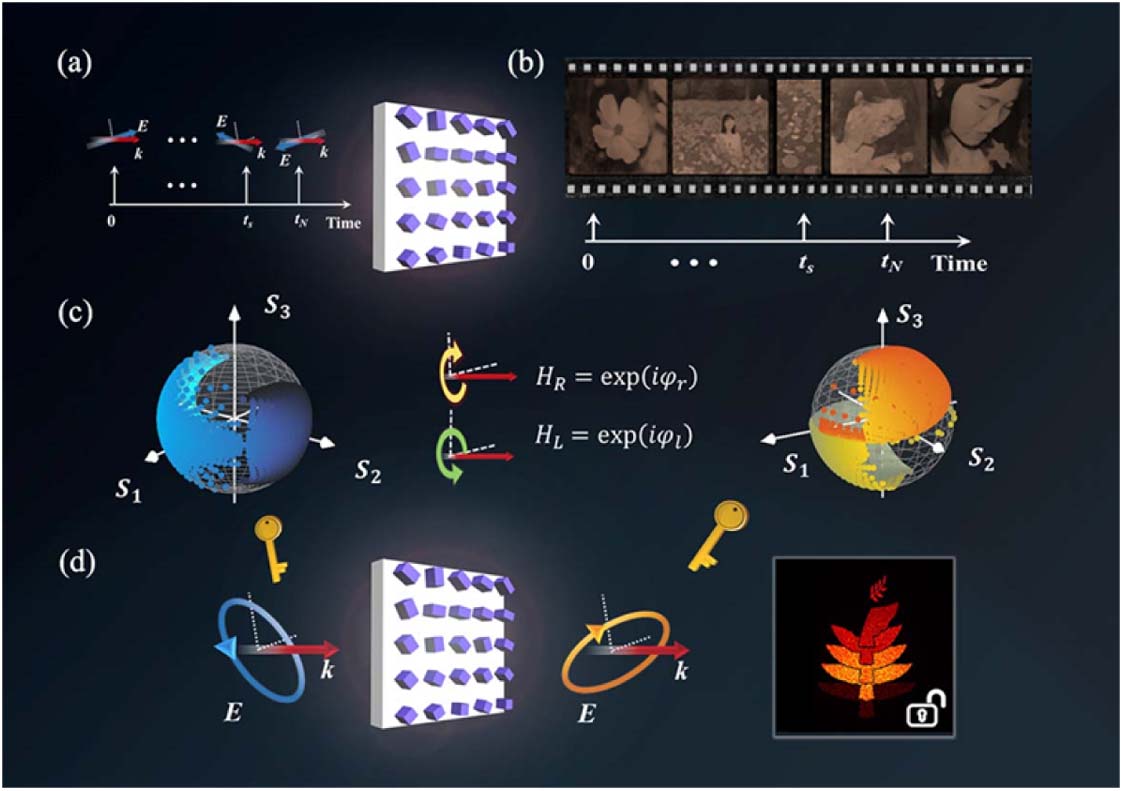
Set citation alerts for the article
Please enter your email address