Shaojie Ma, Biao Yang, Shuang Zhang, "Topological photonics in metamaterials," Photon. Insights 1, R02 (2022)

Search by keywords or author
- Photonics Insights
- Vol. 1, Issue 1, R02 (2022)
![(a) Linear dispersion (light cone) of the 3D “massless” photon ω = ±ck. At the origin of momentum space, i.e., k→=0, there exists a magnetic monopole with the quantized Chern number CL/R = ±2 for left/right circular polarization. (b) Schematic diagram of the geometric Berry phase; the parallel transport on a curved surface will accumulate a geometric phase of Δθ related to the solid angle dΩ. (c) Evolution of EFSs and their Chern numbers with additional chirality and hyperbolicity and the topologically protected surface states on the interface between chiral hyperbolic metamaterial and vacuum. (d) Realizable chiral hyperbolic metamaterial and topologically protected surface wave, whereas a backscattering-immune surface wave propagates across a three-dimensional step. (a) Adapted from [59], (c) from [63], and (d) from [64].](/richHtml/pi/2022/1/1/R02/img_001.png)
Fig. 1. (a) Linear dispersion (light cone) of the 3D “massless” photon ω = ±ck . At the origin of momentum space, i.e., , there exists a magnetic monopole with the quantized Chern number C L/R = ±2 for left/right circular polarization. (b) Schematic diagram of the geometric Berry phase; the parallel transport on a curved surface will accumulate a geometric phase of Δθ related to the solid angle dΩ. (c) Evolution of EFSs and their Chern numbers with additional chirality and hyperbolicity and the topologically protected surface states on the interface between chiral hyperbolic metamaterial and vacuum. (d) Realizable chiral hyperbolic metamaterial and topologically protected surface wave, whereas a backscattering-immune surface wave propagates across a three-dimensional step. (a) Adapted from [59], (c) from [63], and (d) from [64].
![(a) Schematic diagram of WPs and the Fermi arcs. (b) Intrinsic topologically protected Fermi arcs connect two WPs with opposite chiralities. (c), (d) Structure and band topology of the ideal photonic Weyl metamaterial. (e) Experimental EFSs of the topological helicoidal surface states. (f), (g) A pseudo-gauge field generated by the space-dependent rotation angle supports a zeroth-order chiral Landau level with one-way propagation in ideal Weyl semimetals. (h) Dispersion spectrum of plasmonic WPs in magnetized plasma with time-reversal symmetry (TRS) broken. (i) Unit structure and EFS of an ideal unconventional Weyl semimetal in a chiral photonic metamaterial with C = ±2. (c)–(e) Adapted from [76,77], (f), (g) from [54], (h) from [78], and (i) from [79].](/richHtml/pi/2022/1/1/R02/img_002.png)
Fig. 2. (a) Schematic diagram of WPs and the Fermi arcs. (b) Intrinsic topologically protected Fermi arcs connect two WPs with opposite chiralities. (c), (d) Structure and band topology of the ideal photonic Weyl metamaterial. (e) Experimental EFSs of the topological helicoidal surface states. (f), (g) A pseudo-gauge field generated by the space-dependent rotation angle supports a zeroth-order chiral Landau level with one-way propagation in ideal Weyl semimetals. (h) Dispersion spectrum of plasmonic WPs in magnetized plasma with time-reversal symmetry (TRS) broken. (i) Unit structure and EFS of an ideal unconventional Weyl semimetal in a chiral photonic metamaterial with C = ±2. (c)–(e) Adapted from [76,77], (f), (g) from [54], (h) from [78], and (i) from [79].
![(a) Unit of an I-shaped metamaterial satisfying the space group P4/mbm (No. 127). (b) Simulated band structure along high symmetry lines. (c) The measured bulk and surface dispersions identify a single nodal ring in the metamaterial. (d)–(f) Similar to (a)–(c), but for another metamaterial configuration satisfying space group P4/nbm (No. 125). (g) Biaxial hyperbolic metamaterial belonging to space group Pmmm (No. 47). (h) Nodal link structure in the metamaterial. (i) Graphical representation of the quaternion group Q and associated non-Abelian group multiplications. (a)–(c) Adapted from [108], (d)–(f) from [109], (g), (h) from [110], and (i) from [111].](/Images/icon/loading.gif)
Fig. 3. (a) Unit of an I-shaped metamaterial satisfying the space group P 4/mbm (No. 127). (b) Simulated band structure along high symmetry lines. (c) The measured bulk and surface dispersions identify a single nodal ring in the metamaterial. (d)–(f) Similar to (a)–(c), but for another metamaterial configuration satisfying space group P 4/nbm (No. 125). (g) Biaxial hyperbolic metamaterial belonging to space group Pmmm (No. 47). (h) Nodal link structure in the metamaterial. (i) Graphical representation of the quaternion group and associated non-Abelian group multiplications. (a)–(c) Adapted from [108], (d)–(f) from [109], (g), (h) from [110], and (i) from [111].
![(a) Schematic of the 3D photonic Dirac metamaterial, with each unit cell consisting of eight helical elements satisfying D4h point symmetry. (b) Dispersions along ky (kz). (c) Spin-dependent Fermi arcs identify the DP in such a metamaterial. (d) Modified 5D YM metamaterial with introducing additional synthetic k4 and k5 dimensions and 5D k-space (3D k-subspace) distributions of the linked WSs perturbed from the YM metamaterial. (e) 1D Weyl arcs for both a system with YM and that with Weyl surfaces. (f) Simulated and measured dispersions of surface states along kz direction for YM metamaterial. (a)–(c) Adapted from [119,120] and (d)–(f) from [121].](/Images/icon/loading.gif)
Fig. 4. (a) Schematic of the 3D photonic Dirac metamaterial, with each unit cell consisting of eight helical elements satisfying D 4h point symmetry. (b) Dispersions along k y (k z ). (c) Spin-dependent Fermi arcs identify the DP in such a metamaterial. (d) Modified 5D YM metamaterial with introducing additional synthetic k 4 and k 5 dimensions and 5D k -space (3D k -subspace) distributions of the linked WSs perturbed from the YM metamaterial. (e) 1D Weyl arcs for both a system with YM and that with Weyl surfaces. (f) Simulated and measured dispersions of surface states along k z direction for YM metamaterial. (a)–(c) Adapted from [119,120] and (d)–(f) from [121].
![(a) Band structures along high symmetry lines for (left panel) 3D gapless DP and (right panel) gapped TI, where the complete bandgap is shadowed. Inset: corresponding photonic metamaterial unit with/without breaking Mz mirror symmetry. (b) Experimental demonstration of the robustness of photonic surface states between TIs with the opposite mass terms. (c) Similar to (a), but for a 3D NL and fully gapped NL exhibiting Berry curvature vortex. (d) Experimentally mapped interface states between back-to-back MTMs. (a), (b) Adapted from [130] and (c), (d) from [131].](/Images/icon/loading.gif)
Fig. 5. (a) Band structures along high symmetry lines for (left panel) 3D gapless DP and (right panel) gapped TI, where the complete bandgap is shadowed. Inset: corresponding photonic metamaterial unit with/without breaking M z mirror symmetry. (b) Experimental demonstration of the robustness of photonic surface states between TIs with the opposite mass terms. (c) Similar to (a), but for a 3D NL and fully gapped NL exhibiting Berry curvature vortex. (d) Experimentally mapped interface states between back-to-back MTMs. (a), (b) Adapted from [130] and (c), (d) from [131].
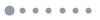
Fig. 6. Illustration of the topological phase transition on the metamaterial platform.
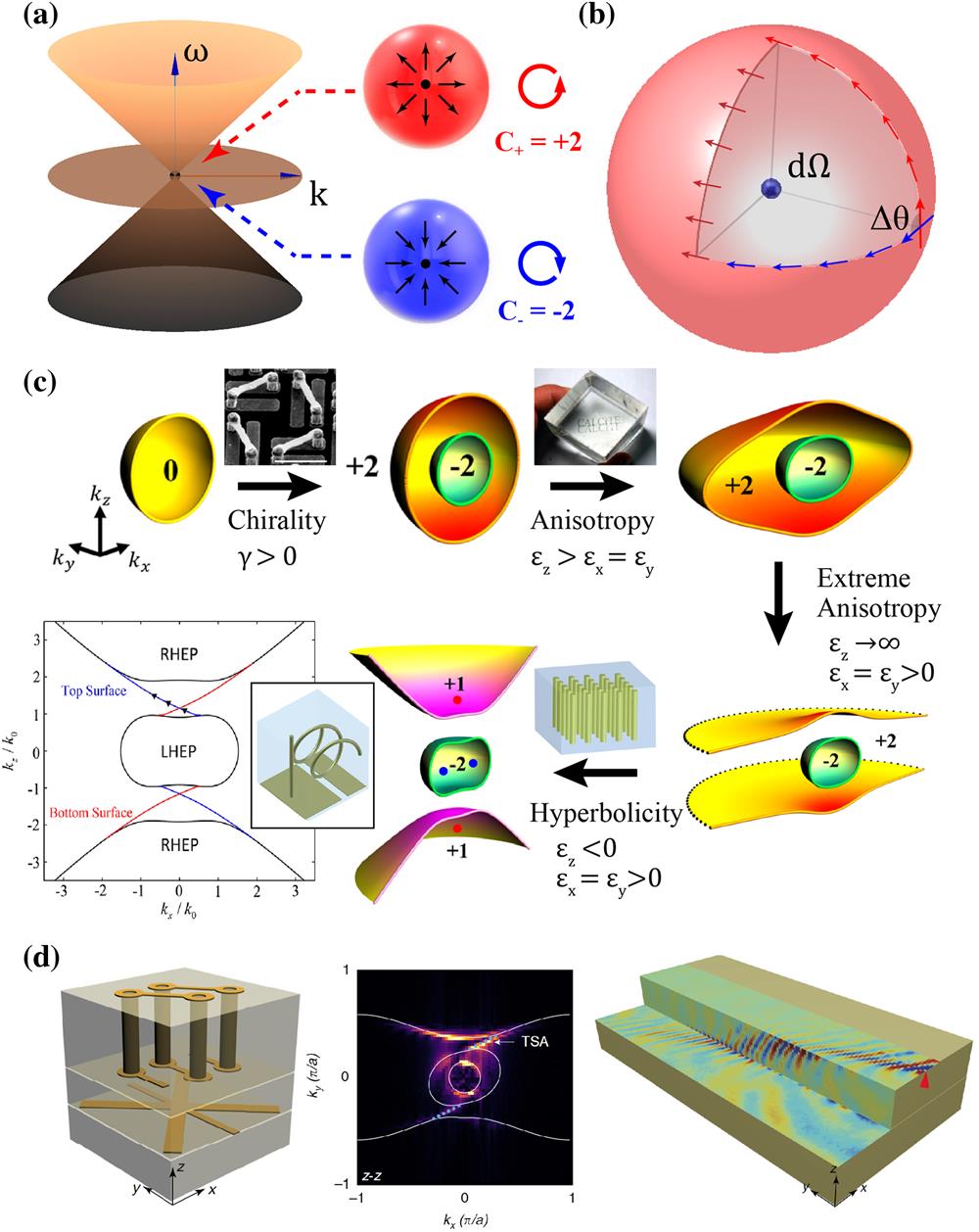
Set citation alerts for the article
Please enter your email address