Author Affiliations
1Zhejiang University, College of Optical Science and Engineering, New Cornerstone Science Laboratory, Interdisciplinary Center for Quantum Information, Hangzhou, China2Jiaxing Institute of Zhejiang University, Intelligent Optics and Photonics Research Center, Jiaxing, China3Shanxi University, Collaborative Innovation Center of Extreme Optics, Taiyuan, Chinashow less
Fig. 1. Schematic illustration of the CNP waveguiding scheme. (a) Freestanding CNP waveguide, coupled by a fiber taper drawn from a standard glass fiber. (b) On-chip CNP waveguide, coupled by a tapered planar waveguide.
Fig. 2. Four lowest eigenmodes of a CNP waveguide. Normalized electric field distribution (left panel) and surface polarized bound charge density distribution (right panel) of (a) -like, (b) -like, (c) -like, and (d) -like modes in a CdS CNP with a nanowire diameter of 180 nm at 550-nm wavelength. The scale bar in (a) applies to (b)–(d). (e)–(h) Diameter-dependent of the four lowest modes of (e) a freestanding CNP at 550-nm wavelength, (f) a freestanding CNP at 750-nm wavelength, (g) an on-chip CNP at 550-nm wavelength, and (h) an on-chip CNP at 650-nm wavelength. The blue-shaded areas represent the selected diameter areas in this work. The dashed black lines indicate the refractive index of the substrate.
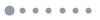
Fig. 3. Mode evolution in a freestanding CdS CNP waveguide. (a) Schematic diagram of the coupling structure. A horizontal coordinate indicates the waveguide length originated from the left end of the CNP. The blue dashed line indicates the obscured profile of the fiber taper beneath the CNP. (b)–(e) Field intensity distribution in planes (cross-sectional planes) at corresponding positions marked by yellow arrows. The simulation is carried out with a nanowire diameter of 140 nm and a fiber-taper angle of 3 deg at 650-nm wavelength. The insets in (d) and (e) are close-up views of the field intensity around the CNP. The scale bar in (b) applies to (c)–(e). (f) of the mode of the fiber taper and the nanoslit -like mode of the CNP within the spectral range of 550 to 750 nm. The blue and pink areas indicate the nanoslit mode and the mode, respectively. The intersecting red area is the -matching area of the two modes within the spectral range of 550 to 750 nm, and the solid yellow line represents the matched of the two modes at the same wavelength with respect to the fiber taper diameter (see Sec. S4 in the Supplemental Material). (g) Broadband coupling efficiency and mode purity of the -like nanoslit mode.
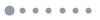
Fig. 4. Sub-nm-confined optical fields in the nanoslit mode at visible spectrum. (a) Normalized cross-sectional field intensity distribution of the -like nanoslit mode of a CdS CNP with , , , and . The coordinate origin is located at the center of the slit. (b) FWHM of the field intensity of the -like mode along the axis (blue line) and axis (orange line) with . (c) -dependent FWHM of the field intensity along the axis (blue line) and axis (orange line). (d) Power density (Poynting vector in the direction, ) distribution around the slit cross section. The closed white line indicates the contour of (0, 0)/2, marking the profile of the central hot spot. (e) Overall distribution around the CNP cross section in a log scale. The closed white line indicates the boundary of the effective mode area. The units of all coordinates of (d) and (e) are nanometers. (f) 3-D plot of the normalized cross-sectional field intensity distribution of the -like nanoslit mode. (g) Field intensity distribution along the -axis direction. For better clarity, a profile is also plotted as dotted lines. (h) -dependent peak-to-background ratio (blue line) and (orange line) of the -like nanoslit mode, respectively.
Fig. 5. Sub-nm-confined optical fields in the nanoslit mode at MIR spectrum. (a) Normalized cross-sectional field intensity distribution of the -like nanoslit mode of a CdS CNP with , , , and . (b) -dependent FWHM of the field intensity along the axis (solid line) and axis (dashed line) of a CdS CNP and a CdTe CNP with the same geometries, respectively. (c) distribution around the slit cross section. The closed white line indicates the contour of (0, 0)/2, marking the profile of the central hot spot. (d) Overall distribution around the CNP cross section in a log scale. The closed white line indicates the boundary of the effective mode area. The units of all coordinates of (c) and (d) are nanometers. (e) -dependent peak-to-background ratio (solid line) and (dashed line) of the -like nanoslit mode of CdS and CdTe CNPs, respectively.
Fig. 6. -dependent and of the nanoslit mode in a CdS CNP at (a) visible spectrum with and (b) MIR spectrum with . In all calculations, is assumed to be 1 nm and is assumed to be 5 nm.