Author Affiliations
1Xi’an Jiaotong University, School of Electronic Science and Engineering, Key Laboratory for Physical Electronics and Devices of the Ministry of Education & Shaanxi Key Lab of Information Photonic Technique, Faculty of Electronic and Information Engineering, Xi’an, China2Nankai University, TEDA Applied Physics Institute and School of Physics, MOE Key Laboratory of Weak-Light Nonlinear Photonics, Tianjin, China3San Francisco State University, Department of Physics and Astronomy, San Francisco, California, USAshow less
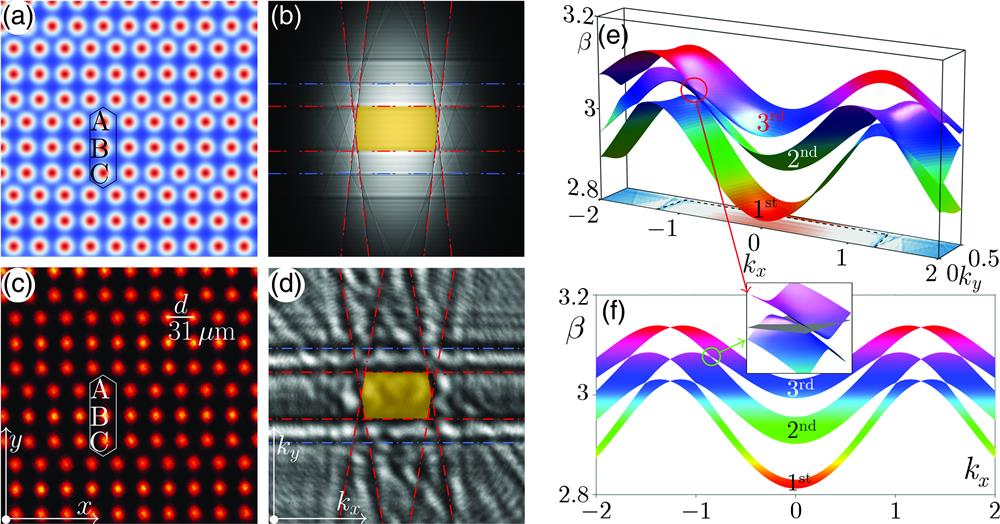
Fig. 1. Photonic lattices with type-II Dirac cones. (a) Numerically designed lattice structure with , , and marked as the three sites in a unit cell indicated by a hexagon. Red spots represent the lattice sites. (b) BZ spectrum of (a) with the red dashed lines contouring the first BZ (shaded region). (c), (d) Experimental realization of the lattice and its BZ spectrum measured with the white-light BZ spectroscopy technique, corresponding to (a) and (b). In panel (c), bright spots represent the lattice sites. (e) Band structure of the photonic lattice in which type-II Dirac points exist due to the tilted band-touching between the first and second bands, and second and third bands. The projected BZ is shown in the plane. (f) Projection of the band structure in the plane. The inset is a zoom-in plot of the type-II Dirac cone indicated by circles with arrows. The gray horizontal plane in coordinates goes across the Dirac point and intersects with the Dirac cone to form a pair of crossing lines—a direct manifestation of the type-II Dirac cone.
Fig. 2. Linear topological VHE states at the DW between two type-II Dirac cone photonic lattices. (a) and (b) Inversion-symmetry-broken photonic lattices with the depth of site C and site A increased, respectively. (c) Band structure corresponding to the lattice in (a) [which looks the same for the lattice in (b)]. (d) Berry curvature of the first band displayed in the plane. The top panel corresponds to (a); the bottom panel, which is opposite, corresponds to (b). The dashed hexagon represents the first BZ. (e) A DW is established by combining (a) and (b) with an interface, highlighted by a green rectangle. The lattice is periodic along the direction but has boundaries along the direction. (f) Band structure of the lattice in (e); the red curve represents the topologically protected VHE state along the DW. In panels (a), (b), and (e), blue and red spots represent the lattice sites with different detunings.
![Numerically obtained nonlinear topological VHE states and robust transport of quasi-solitons from MI. (a) Dispersion spectrum of the linear edge state in Fig. 2(f). Solid curve is for β′ and dashed curve for β″. VHE quasi-solitons are found only in the region with β″>0. (b) Plots of amplitude a and power P of the nonlinear edge states at kx=0.1K versus μ. The red dotted line corresponds to μ=2.736. (c) Profiles of a typical nonlinear edge state (superimposed with 5% random noise) found at μ=2.736 at z=0 and z=1750. (d) Amplitude of the nonlinear state in (c) versus propagation distance. (e) Amplitude a (red curve) and barycenter xc (blue curve) of a quasi-soliton filament [marked by the red circle in (c)] resulting from MI, showing the robustness of the quasi-soliton over extremely long distances, as seen also in the intensity pattern snapshots (f) during transport. (g) Spreading of the same input at z=0 as in (f) along the DW during linear propagation for comparison. (Here, z=1000 corresponds to a physical distance 4 m.)](/Images/icon/loading.gif)
Fig. 3. Numerically obtained nonlinear topological VHE states and robust transport of quasi-solitons from MI. (a) Dispersion spectrum of the linear edge state in Fig. 2(f). Solid curve is for and dashed curve for . VHE quasi-solitons are found only in the region with . (b) Plots of amplitude and power of the nonlinear edge states at versus . The red dotted line corresponds to . (c) Profiles of a typical nonlinear edge state (superimposed with 5% random noise) found at at and . (d) Amplitude of the nonlinear state in (c) versus propagation distance. (e) Amplitude (red curve) and barycenter (blue curve) of a quasi-soliton filament [marked by the red circle in (c)] resulting from MI, showing the robustness of the quasi-soliton over extremely long distances, as seen also in the intensity pattern snapshots (f) during transport. (g) Spreading of the same input at as in (f) along the DW during linear propagation for comparison. (Here, corresponds to a physical distance 4 m.)
![Experimental observation of nonlinear topological VHE states. (a1) Experimentally established type-II Dirac lattices with a center DW (marked by the white line), where the inset (bottom-right) shows discrete diffraction from single-site excitation. (b)–(d) Linear (first and third rows) and nonlinear (second and fourth rows) outputs in real (first and second rows) and momentum (third and fourth rows) space obtained from excitation by two superimposed out-of-phase elliptical beams (circled by the dashed ellipse). The initial Bloch momenta of the probe beam are (b) −3π/5d, (c) 0, and (d) 3π/5d, as marked by colored triangles in the spectra, where the white dots mark the BZ center for reference. The first two rows are presented under the same color scale as shown in (d1) and (d2). Solid ellipses in the top two rows mark the portion in the input beam that is “diminished” by nonlinearity. (e) Dashed (linear) and solid (nonlinear) curves illustrate the relative position of normalized intensity profiles of the output beam along the x axis, and vertical lines mark the corresponding center position of the beam. White dashed curve in (e1) corresponds to the input beam for reference. Shifting of the beam position in (b2) [e(2)] and (d2) [(e4)] signals the nonlinearity-induced transport of the VHEs, as analyzed in our theory and corroborated by numerical simulations.](/Images/icon/loading.gif)
Fig. 4. Experimental observation of nonlinear topological VHE states. (a1) Experimentally established type-II Dirac lattices with a center DW (marked by the white line), where the inset (bottom-right) shows discrete diffraction from single-site excitation. (b)–(d) Linear (first and third rows) and nonlinear (second and fourth rows) outputs in real (first and second rows) and momentum (third and fourth rows) space obtained from excitation by two superimposed out-of-phase elliptical beams (circled by the dashed ellipse). The initial Bloch momenta of the probe beam are (b) , (c) 0, and (d) , as marked by colored triangles in the spectra, where the white dots mark the BZ center for reference. The first two rows are presented under the same color scale as shown in (d1) and (d2). Solid ellipses in the top two rows mark the portion in the input beam that is “diminished” by nonlinearity. (e) Dashed (linear) and solid (nonlinear) curves illustrate the relative position of normalized intensity profiles of the output beam along the axis, and vertical lines mark the corresponding center position of the beam. White dashed curve in (e1) corresponds to the input beam for reference. Shifting of the beam position in (b2) [e(2)] and (d2) [(e4)] signals the nonlinearity-induced transport of the VHEs, as analyzed in our theory and corroborated by numerical simulations.