Abstract
Photoluminescence (PL) bands from potassium dihydrogen phosphate (KDP) crystals are studied by time-resolved PL spectroscopy. KDP crystals irradiated at a laser fluence of 11.5 J/cm2 are found to have the highest probability of phosphorus–oxygen hole center defects and the lowest probability of phosphorus-oxygen electric center defects, in contrast to the probabilities of these defects for KDP crystals irradiated at 9.0 J/cm2. The probabilities of these two defects occurring in retired components are found to be intermediate between those for crystals irradiated at the two different fluences. The two types of defects may result from two different mechanisms and may interconvert under certain conditions. Thus, there are differences between the defects in KDP crystals irradiated at a high laser fluence and those in retired components.
I. INTRODUCTION
Potassium dihydrogen phosphate (KH2PO4 or KDP) crystals are widely used in nonlinear optics owing to their high-frequency conversion qualities and because they can be grown rapidly. They are important in large laser systems, such as the National Ignition Facility in the USA.1 However, crystals in such high-power systems are subject to laser-induced damage (LID), which limits the output of the system.2,3
LID arises from defects or impurities created during the crystal growth process. The most common defects in KDP crystals are related to the hydrogen bonds among the PO4 groups. Many studies have reported two kinds of defects that are caused by hydrogen bonding, namely, A and B radicals. Duchateau et al.4 reported the formation of B radicals and self-trapped excitation defects in KDP and deuterated KDP (DKDP) crystals irradiated by femtosecond laser pulses at 800 nm, whereas A and B radical defects were formed at wavelengths under 266 nm.5 Ogorodnikov et al.6–9 showed that the luminescence spectrum at 5.1 eV originates from the radiative annihilation of self-trapped excitons (STEs). These defects may participate in ionization processes and lead to the formation of a plasma, resulting in LID.10 Although defects in KDP crystals have been extensively studied, the evolution of defects in laser-irradiated KDP crystals remains poorly understood.
Time-resolved photoluminescence (PL) spectroscopy is a sensitive method for defect detection and can also be used to obtain the decay lifetime. This method is able to provide real-time information on microscopic structural changes in KDP crystals. There have been a number of reports of PL properties and structural defects in KDP crystals,11–15 although defect evolution has rarely been studied. In the present work, the evolution of defects was investigated through time-resolved PL spectroscopy both in KDP crystals subjected to laser irradiation at high fluences and in retired components. The defects in the laser-irradiated KDP crystals and the retired components were then compared. The samples and experimental procedure are described in Sec. II. Results are presented and discussed in Sec. III. Conclusions are summarized in Sec. IV. This work shows that the probabilities of defects appearing in retired components are different to those appearing in KDP crystals irradiated by lasers at high fluences.
II. SAMPLES AND EXPERIMENTS
Some of the samples were retired components. These were irradiated by 400 pulses. The average and peak fluences were 1.2 J/cm2 and 1.8 J/cm2, respectively. These crystals were of dimensions 10 × 20 × 1 mm3. The remaining KDP crystals were irradiated with fluences of 9.0 J/cm2 and 11.5 J/cm2. These crystals were of dimensions 10 × 10 × 1 mm3. The sample and laser parameters are discussed in detail in Ref. 16.
PL signals from the samples were collected using a custom-built micro-PL system. The time-integrated PL spectra were measured using a spectrometer (MS2004i, SOL Instruments) equipped with a 1200 lines/mm grating (with a spectral resolution of 0.4 nm). A 405 nm picosecond diode laser (PiLas, A.L.S. GmbH) was used for excitation. The fast signals were detected and recorded by a microchannel plate photomultiplier tube (R3809U-50, Hamamatsu) with a high-speed amplifier (C5594 series, Hamamatsu) and a time-correlated single-photon counting module (TCSPC) board (SPC-130, Becker & Hickl GmbH). The lifetime spectra were also measured using a TCSPC module (SPC-130). In the experiments, the 400 nm and 800 nm PL spectral regions were explored.
III. RESULTS AND DISCUSSION
The spectrum of the light source with 405 nm excitation wavelength is shown by curve (a) in Fig. 1, while curves (a) and (b) are PL emission spectra from a KDP crystal at different damage sites. The emission spectra from both sites are in the form of a broad band ranging from 400 nm to 800 nm and centered at 520 nm. However, differences can be observed between these emission spectra, which suggests that the defects might have different origins.
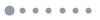
Figure 1.(a) Spectrum of light source. (b) and (c) PL emission spectra from a KDP crystal at different damage sites.
Figure 2 shows experimental and simulated spectra, together with Gaussian fits. The excitation light source spectrum [Fig. 2(a)] consists of two peaks: A1 and A2. In the emission spectra from the KDP crystal at two different damage sites [Figs. 2(b) and 2(c)], the peaks on the high-energy side are the same as those in the source spectrum and thus are independent of the crystal structure. On the low-energy side, however, there are clear differences between the spectra from the two damage sites, and it can be assumed that the different bands in these spectra arise from different types of defects. It is found that each type of defect can be successfully fitted by its own Gaussian curve with the parameters shown in Table I.
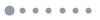
Figure 2.Experimental spectra (black solid curves), simulated spectra (red dashed curves), and Gaussian fits (blue dashed curves): (a) excitation light source; (b) and (c) PL emission spectra of a KDP crystal at different damage sites.
| Position | Area (a.u.) | FWHM (nm) |
---|
Peak | a | b | c | a | b | c | a | b | c |
---|
A1 | 417.92 | 418.43 | 418.08 | 4.25 | 4.40 | 4.13 | 5.27 | 6.12 | 5.75 |
A2 | 424.10 | 426.66 | 426.04 | 4.41 | 6.38 | 3.99 | 13.68 | 20.85 | 14.16 |
B | | 467.96 | 473.49 | | 15.16 | 17.59 | | 52.87 | 76.05 |
C | | 510.95 | 512.91 | | 9.56 | 14.11 | | 46.44 | 58.64 |
D | | 550.50 | 560.09 | | 15.68 | 22.00 | | 73.81 | 79.51 |
E | | 587.89 | | | 29.70 | | | 152.81 | |
F | | | 635.14 | | | 34.22 | | | 216.69 |
Table 1. Parameters of peaks with Gaussian fitting for samples irradiated by different fluences.
Müller17 identified several intrinsic lattice defects in KDP crystals by electron paramagnetic resonance spectroscopy. The two simple defects are vacant (L defect) and twice-occupied (D defect) hydrogen bonds. A considerable number of L defects are formed during crystal growth. The H2PO4− group loses a proton of the hydrogen bond and forms an A radical, which is a hole-trapping center. This group is the closest to the hydrogen vacancy and is located at the oxygen atom. The A radical can be formed in two ways. The first is related to the L defect and forms as follows: L + h+ → L+ ≡ A. The second results in holes after laser radiation.
A hydrogen ion captures an electron and transforms into a neutral hydrogen atom. This atom is then pushed outside the regular lattice site. The closest oxygen atom to the vacancy captures a hole and forms an A radical. When the neutral hydrogen atom attaches at a free position of a hydrogen bond, an H3PO4 group (D defect) is formed.18 Through electron paramagnetic resonance, Setzler et al.19 found that the concentration of the A radical corresponds to that of neutral hydrogen atoms. The B radical is a self-trapped hole, and its formation is accompanied by the formation of an electron-trapping center. Interstitial hydrogen ions are common electron-trapping centers in KDP crystals.
Previous studies have shown that radiation induces defects in phosphate glasses.20–22 Several color centers have been determined, and these are responsible for absorption bands. However, the PL bands associated with these defects have rarely been discussed in the literature. In Fig. 2, the PL band at 467 nm is associated with an L defect, which, according to Ogorodnikov et al.,23 become an A radical when a hole is localized. This band plays an important role in the optical properties of KDP crystals and has therefore been the subject of intensive studies. Green PL bands (400–800 nm) are commonly reported at LID sites. These bands can be assigned to well-known defects. The 510 nm PL band has been attributed to electron–hole recombination close to the PO4 group.24 The 560 nm PL band is attributable to an A radical. The broad PL band centered at 587 nm has been attributed to a laser-induced phosphorus–oxygen hole center (POHC) defect.25 This defect is a typical result of laser irradiation damage in the phosphate network.26 The origin of the 635 nm PL defect has rarely been considered in the literature. The present study proposes that this defect may be the result of a phosphorus–oxygen electric center (POEC). This assignment is supported by the presence of a similar defect in fused silica. Fused silica and KDP have similar atomic structures and exhibit spectroscopic similarities. PL spectra were obtained from KDP crystals at different damage sites, and the associated defects were found to be different from those associated with the spectra shown in Fig. 2. Spectral parameters were obtained by fitting to Gaussian curves. The bands were found to consist of several overlapping components.
The PL spectra of both retired components and KDP crystals irradiated at 9.0 J/cm2 and 11.5 J/cm2 were measured, and hundreds of sites with PL signals were found at each fluence. Table II shows the statistical results for the PL bands. Similar fluorescence behavior was found for all the samples. The defects are assumed to be related to the H2PO4− group. The 467 nm, 510 nm, and 560 nm bands appear in the damage craters, whereas the 587 nm and 635 nm PL bands appear at other sites. The retired components have medium, long, and short chains, whereas only short and medium chains are found in crystals irradiated with 9.0 J/cm2 and 11.5 J/cm2, respectively.16
| B | C | D | E | F |
---|
Position (nm) | 467 | 510 | 560 | 587 | 635 |
9.0 J/cm2 fluence (%) | 100 | 92.9 | 100 | 52.0 | 29.6 |
Retired (%) | 100 | 94.0 | 100 | 54.1 | 13.5 |
11.5 J/cm2 fluence (%) | 100 | 98.8 | 100 | 85.5 | 2.40 |
Table 2. Probabilities of different types of defects.
We have defined the probability of a particular type of defect as the percentage of the number of such defects to the total number of defects measured during the experiment. Table II shows that the probability of POHC defects in KDP crystals irradiated at a laser fluence of 11.5 J/cm2 is 85.5%, whereas the probabilities of these defects in retired components and crystals irradiated at 9.0 J/cm2 are 54.1% and 52.0%, respectively. By contrast, the probability of POECs in KDP crystals irradiated at a laser fluence of 11.5 J/cm2 is 2.4%, and the probabilities in retired components and crystals irradiated at 9.0 J/cm2 are 13.5% and 29.6%, respectively. These defects may result in two different, but interchangeable, ways. The POHC defect is primarily present in medium-chain structures, because oxygen bridges are formed in long-chain structures. These bridges increase the lengths of P–O bonds and thus facilitate the formation of POHC defects. POECs occur primarily in short-chain structures following the loss of –OH groups. Thus, KDP crystals irradiated at a laser fluence of 11.5 J/cm2 have the highest probability of POHC defects and the lowest probability of POECs. This is in contrast to what is found for KDP crystals irradiated at 9.0 J/cm2. The probabilities of these two defects occurring in retired components are intermediate between the probabilities for KDP crystals irradiated at the two different fluences. This is primarily due to the inhomogeneous nature of the damages to the retired samples and to the low fluence below 400 pulses. These two types of defects interconvert under certain conditions. This led to the differences between the defects in the KDP crystals irradiated at a high laser fluence and those in the retired components.
Irradiation leads to electron transitions from the ground state to an excited state. These excited electrons then return to the ground state by relaxation or radiation. If the rates of radiative and nonradiative transition are denoted by Γ and knr, respectively, the decay rate is given bywhere n(t) is the number of electrons in the excited state, and Γ and knr are the rates of radiative and nonradiative transition, respectively. The decay equation is thenwhere τ is the decay lifetime. Given that the fluorescence intensity is proportional to the number of decaying electrons, the PL spectra of the PL bands show that the initial intensity is Ik(λ) at wavelength λ.27 The decay lifetime τk for the kth PL band is a single exponential, and the signal S(λ, td) at a time delay td can then be described as follows:The lifetime, band width, and initial intensity of each PL band can be analyzed by multi-exponential fitting with different decay times. The number of overlapping PL bands is important, because it can be used to determine the number of fitting parameters in the multi-exponential fit. When the number of the PL bands has been confirmed, the intensity Ik(λ) and lifetime τk of each band can be found by multi-exponential fitting of the bands in accordance with Eq. (3).
Figure 3 depicts the emission decay curves at 405 nm excitation energy. These curves can be effectively fitted by a double-exponential function.
I(t) = I1 exp(−t/τ1) + I2 exp(−t/τ2) + I0, where τ1 and τ2 are the lifetimes (in the nanosecond range) in our experiment. The lifetimes at 600 nm represent the same decay kinetics. Comparison of the spectra in Figs. 2(b) and 2(c) reveals that the peak C is shared by the overlapping PL bands at 600 nm. Table III shows the fitting parameters for a double-exponential fit of the PL decay kinetics at 600 nm. The lifetime τ1 of the A radical is ∼4 ns. The lifetimes of the POHC and POEC defects are 0.74 ns and 0.04 ns, respectively. The double-exponential lifetimes may result from the structural properties of the host lattice and the presence of two different types of defect in the crystal.
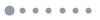
Figure 3.PL decay kinetics at 600 nm of a KDP crystal. The plots labeled (b) and (c) correspond to the spectra in Figs. 2(b) and 2(c), respectively. The black solid curves are the experimental spectra, and the red dashed curves are double-exponential simulated spectra.
| I1 | τ1 (ns) | I2 | τ2 (ns) |
---|
b | 0.50 | 4.04 | 0.61 | 0.74 |
c | 0.55 | 4.23 | 2.69 | 0.04 |
Table 3. Parameters of PL decay kinetics at 600 nm.
IV. CONCLUSIONS
The origin of PL bands from KDP crystals at different damage sites has been studied on the basis of the combined lifetime and intensity spectra from time-resolved PL spectroscopy. Overlapping PL bands in the KDP crystals have been investigated in detail. A lifetime spectrum method has been introduced to efficiently resolve strongly overlapping PL bands. The broad PL band at 587 nm is attributable to a laser-induced POHC defect. The 635 nm PL defect may be the result of a POEC defect. The probabilities of these two types of defects occurring in retired components are intermediate between the probabilities of their occurrence in KDP crystals irradiated at different laser fluences. These defects may result from two different mechanisms and may interconvert under certain conditions. As a consequence there are differences between the defects in KDP crystals irradiated at a high laser fluence and those in retired components.
References
[1] A. K. Burnham, J. J. De Yoreo, P. K. Whitman. Developing KH2PO4 and KD2PO4 crystals for the world’s most power laser. Int. Mater. Rev., 47, 113(2002).
[2] P. Bräunlich, P. Kelly, A. Schmid. Optical breakdown in alkali halides. Phys. Rev. B, 16, 4569(1977).
[3] S. Backus, H. Kapteyn, G. Mourou, M. Murnane, A.-C. Tien. Short-pulse laser damage in transparent materials as a function of pulse duration. Phys. Rev. Lett., 82, 3883(1999).
[4] G. Duchateau, A. Dyan, G. Geoffroy, S. Guizard, H. Piombini. Electron-hole dynamics in normal and deuterated KH2PO4 illuminated by intense femtosecond laser pulses. Phys. Rev. B, 83, 075114(2011).
[5] A. Belsky, G. Duchateau, N. Fedorov, G. Geoffroy, S. Guizard, P. Martin. Interaction of intense femtosecond laser pulses with KDP and DKDP crystals in the short wavelength regime. J. Phys.: Condens. Matter, 25, 435501(2013).
[6] I. N. Ogorodnikov, V. A. Pustovarov et al. Low-temperature time-resolved vacuum ultraviolet luminescent spectroscopy of KH2PO4 crystals with defects. Opt. Spectrosc, 91, 224-231(2001).
[7] V. S. Cheremnykh, M. Kirm, I. N. Ogorodnikov, V. A. Pustovarov. Low-temperature time-resolved vacuum ultraviolet spectroscopy of self-trapped excitons in KH2PO4 crystals. Opt. Spectrosc, 95, 385-389(2003).
[8] V. S. Cheremnykh, M. Kirm, I. N. Ogorodnikov, V. A. Pustovarov. A time-resolved luminescence spectroscopy study of self-trapped excitons in KH2PO4 crystals. Radiat. Meas., 38, 331(2004).
[9] I. N. Ogorodnikov, M. K. Satybaldieva, B. V. Shul’gin, V. Yu. Yakovlev. Transient optical absorption of hole polarons in ADP (NH4H2PO4) and KDP (KH2PO4) crystals. Phys. Solid State, 44, 880(2001).
[10] P. DeMange, S. G. Demos, M. D. Feit, R. A. Negres. Investigation of the electronic and physical properties of defect structures responsible for laser-induced damage in DKDP crystals. Opt. Express, 18, 13788(2010).
[11] R. Dai, Y. Li, C. Tong, C. Xu, L. Yang, Y. Zhu. Mineralization and optical properties of Eu3+-doped tricalcium silicate soaked in dilute K2HPO4 aqueous solution. Opt. Mater., 85, 32(2018).
[12] R. Ba, B. Chen, L. Ding, J. Li, Y. Li, J. Na, H. Xu, X. Yang, J. Yuan, W. Zheng, Y. Zheng, X. Zhou. Characteristics of precursors responsible for bulk damage initiation in doubler KDP crystal at different wavelengths. Opt. Laser Technol., 96, 196(2017).
[13] Z. Cao, G. Hu, T. Jitsuno, D. Li, X. Liu, Y. Luo, W. Rudolph, Y. Shan, J. Shao, L. Yang, Y. Zhao. Photoluminescence properties of KDP crystals under high power nanosecond laser irradiation. Proc. SPIE, 10339, 103390V(2017).
[14] K. S. Bartwal, G. Bhagavannarayana, G. S. Lodha, S. K. Sharma, Y. Singh, M. K. Tiwari, S. Verma. Investigations of structural defects, crystalline perfection, metallic impurity concentration and optical quality of flat-top KDP crystal. Opt. Mater., 46, 329(2015).
[15] B. Bertussi, C. Gouldieff, F. Guillet, J.-Y. Natoli, F. R. Wagner. Photoluminescence characterization of KH2PO4 crystal: Application to three-dimensional growth-sector identification. Appl. Opt., 53, 3063(2014).
[16] X. Ju, X. Li, B. a. Liu, C. Liu, C. Yan. Investigating the surface electronic structures of retired components and irradiated KDP crystals with different fluences by XANES spectroscopy. Opt. Mater. Express, 8, 816(2018).
[17] K. A. Müller. Electron spin and paramagnetic resonance in KH2PO4 and its isomorphs. Ferroelectrics, 72, 273(1987).
[18] L. B. Harris, G. J. Vella. Direct current conduction in ammonium and potassium dihydrogen phosphate. J. Chem. Phys., 58, 4550(1971).
[19] J. J. DeYoreo, L. E. Halliburton, S. D. Setzler, K. T. Stevens, M. Yan, N. P. Zaitseva. Hydrogen atoms in KH2PO4 crystals. Phys. Rev. B, 57, 2643(1997).
[20] J. W. Fleming, E. J. Friebele, D. L. Griscom, K. J. Long. Fundamental defect centers in glass: Electron spin resonance and optical absorption studies of irradiated phosphorus-doped silica glass and optical fibers. J. Appl. Phys., 54, 3743(1983).
[21] M. E. Archidi, F. Benyaïch, R. Berger, M. Haddad, A. Nadiri. Defect centers in X-irradiated alkali phosphate glasses: EPR studies. Nucl. Instrum. Methods Phys. Res., Sect. B, 116, 145(1996).
[22] P. Ebeling, D. Ehrt, U. Natura. UV Transmission and radiation-induced defects in phosphate and fluoride–phosphate glasses. J. Non-Cryst. Solids, 263-264, 240(2000).
[23] V. T. Kuanyshev, I. N. Ogorodnikov, V. A. Pustovarov, M. K. Satybaldieva, B. V. Shul’gin. Low-temperature time-resolved vacuum ultraviolet luminescent spectroscopy of KH2PO4 crystals with defects. Opt. Spectrosc., 91, 224(2001).
[24] F. Agulló‐López, J. M. Cabrera, E. Diéguez. Optical absorption and luminescence induced by x rays in KDP, DKDP, and ADP. J. Chem. Phys., 81, 3369(1984).
[25] J. W. Chan, J. S. Hayden, T. Huser, D. M. Krol, S. H. Risbud. Fluorescence spectroscopy of color centers generated in phosphate glasses after exposure to femtosecond laser pulses. J. Am. Ceram. Soc., 85, 1037(2002).
[26] Y.-T. Chen, Y. D. Glinka, S.-H. Lin. The photoluminescence from hydrogen-related species in composites of SiO2 nanoparticles. Appl. Phys. Lett., 75, 778(1999).
[27] B. Li, J. Zhou. Origins of a damage-induced green photoluminescence band in fused silica revealed by time-resolved photoluminescence spectroscopy. Opt. Mater. Express, 7, 2888(2017).