Abstract
Metal sulfide Ag2S is an attractive semiconductor due to its excellent physical and chemical property that enable it with wide applications in fields of catalysis, sensing, optoelectronics in past years. In present work, ?18 mm× 50 mm Ag2S ingot was successfully prepared using zone melting method and its thermoelectric (TE) behavior was investigated. Ag2S has standard monoclinic P21/c space group (α-Ag2S phase) below 450 K and transfer to cubic structure (β-Ag2S phase) over this temperature. Ag2S is a n-type semiconductor as the Seebeck coefficient S is always negative due to the Ag interstitial ions in the material that can provide additional electrons. S is about -1200 μV·K-1near room temperature, declines to -680 μV·K -1 at 440 K and finally decreases to ~-100 μV·K -1at β-Ag2S state. The electrical conductivity (σ) of α-Ag2S is almost zero. However, the value sharply jumps to ~40000.5 S·m -1 as the material just changes to β-Ag2S at 450 K and then gradually deceases to 33256.2 S·m -1 at 650 K. Hall measurement demonstrates that carrier concentration nH of Ag2S is suddenly increased from the level of ~10 17 cm-3 to ~1018 cm-3during phase transition. Total thermal conductivity κ of α-Ag2S is ~0.20 W·m -1·K-1 but is ~0.45 W·m-1·K-1of β-Ag2S. Ultimately, a maximum ZT=0.57 is achieved around 580 K that means Ag2S might be a promising middle-temperature TE material.During the past years, metal sulfide Ag2S has attracted much attention due to its excellent physical and chemical properties that enable it with various applications in fields of catalysis, sensing, optoelectronics and so on[1,2,3,4,5,6]. For example, Dong, et al[7] reports Ag2S-nanowire is an ideal candidate for making nano temperature and photoelectric sensors as its photoconductivity is always positive under 532 or 1064 nm laser radiation. Du, et al[8] declares Ag2S Quantum Dots may act as nontoxic carrier for potential in vivo bioimaging. Zhang, et al[9] confirms that the Ag2S Quantum Dots indeed open up the possibility of in vivo anatomical imaging and early stage tumor diagnosis owing to their high emission efficiency in NIR-II imaging window. Besides, Ag2S is also found suitable for solar cell and infrared sensitivity device fabrication attribute to its semiconductor character which has a ~1.0 eV band gap[10]. Recently, it is announced that Ag2S exhibits a fantastic room-temperature ductile behavior. Its compression deformation can reach 50%, the bending variable surpassing 20%, and the stretching variable up to 4.2%. These shape variables are far more than known ceramic and semiconductor materials, and are equivalent to the mechanical properties of some metals. Consequently, Ag2S provides a possibility is quest of producible inorganic semiconductors/ceramics for flexible electronic devices[11].
In order to develop more interesting functions of Ag2S, the authors focus on its potential thermoelectric (TE) behavior according to the concept of Seebeck-Peltier effect[12]. The TE device can supply green and reliable energy by direct conversion of heat into electricity. Thus, it is expected to have wide applications in power generation. The efficiency of a thermoelectric material is usually evaluated by the dimensionless figure of merit ZT, ZT= (S2Tσ)/κ. Where S is Seebeck coefficient, T is absolute temperature, σ is electrical conductivity and κ is thermal conductivity[13]. From the view of this formula, it is obvious that the TE material with ultra-low thermal conductivity is one of a significant factor for high ZT. Based on this recognition, the TE behavior of Ag2S is worthy of study as it has very small thermal conductivity. Wang, et al[14] have fabricated Ag2S ceramic using a solution method and the thermal transport analysis indicates that its total thermal conductivity is 0.4- 0.6 W·m-1·K-1 in range of 300-600 K, which is lower than most solid TE materials. Ultimately, a maximum ZT=0.55 (580 K) is obtained which implies that Ag2S is a promising middle-temperature TE material. In present work, a zone melting method which has the advantage of purifying materials is introduced for Ag2S compound fabrication. Its electrical/thermal transport properties are systematically investigated and the final figure of merit ZT is demonstrated.
1 Experimental
1.1 Ag2S preparation
99.999% high purity Ag and S elements were used as start materials for Ag2S synthesis, they were weighed in accordance with the standard stoichiometric ratio and the total weight was about 60.5 g. The start materials were loaded into a ϕ18 mm quartz ampoule and then sealed with a vacuum less than 10-2 Pa, after that, the quartz ampoule was placed into a 1000 ℃ rocking furnace. After Ag and S totally melted, the rocking system worked at a rate of 20 r/min for 30 min to enhance Ag2S synthesis homogeneity. Ultimately, Ag2S compound was obtained as the furnace was cooled to room temperature naturally. Subsequently, the synthesized Ag2S raw material with the same ampoule was put into a homemade zone melting furnace. The ampoule was supported by a Al2O3 pedestal and a pair of thermal-couples was installed near the bottom for temperature indication. Fig. 1(a) shows the schematic diagram of the zone melting furnace which was heated by a couple of Si-Mo heaters to form a narrow high temperature zone. Fig. 1(b) is the temperature profile along vertical direction, the temperature gradient for Ag2S solidification was about 30-35 ℃/cm. The furnace temperature was controlled at 920 ℃. After Ag2S raw material was melted, the quartz ampoule was lowered down at the speed of 3.0 mm/h until all solution was exhausted. The parameters for Ag2S solidification are summarized in Table 1.
Parameter | Zone melting |
---|
Weight of raw material/g | 60.5 |
Quartz ampoule size/mm | ϕ18
|
Vacuum of quartz ampoule/Pa | <10-2 |
Furnace temperature/℃ | 920 |
Temperature gradient/(℃·cm-1)
| 30-35 |
Lowering speed/(mm·h-1)
| 3.0 |
Table 1. Parameters for Ag2S fabrication
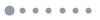
Figure 1.Schematic diagram of the zone melting furnace (a) and temperature profile along vertical direction (b)
1.2 Characterization
The density ρ was measured by Archimedes principle. Phase structure of the material was analyzed by X-ray diffraction (XRD, Bruker D8, Germany) using Cu Kα radiation (λ=0.15406 nm) at room temperature. The morphological and chemical composition were investigated using Scanning Electron Microscope (SEM, JSM-6610, JEOL Ltd.) and Energy Dispersive Spectroscopy (EDS, JED-2300T) equipment. The Seebeck coefficient and electrical conductivity were measured simultaneously (ULVAC-RIKO ZEM-3) from 300 to 650 K. The thermal diffusivity D was tested by laser flash method (Netzsch, LFA-457, Germany). The total thermal conductivity κ was obtained using κ=D·ρ·Cp, where Cp is specific heat capacity.
2 Results and discussion
The as-grown Ag2S ingot (ϕ18 mm× 50 mm) is easily separated from quartz ampoule and displays bright metallic luster, as Fig. 2 shows. Such phenomenon indicates that Ag2S has none reaction with quartz ampoule during the whole process. Its density is measured to be 7.20 g/cm3 that is nearly 100% close to the theoretical value 7.23 g/cm3. Fig. 3(a) is the XRD pattern of Ag2S powder, it is observed that all diffraction peaks are matched well to those of standard α-Ag2S monoclinic P21/c space group (PDF#14-0072) at room temperature. The lattice parameters a, b and c are calculated via a general structure analysis system, and the values are 0.4251, 0.6962 and 0.7873 nm, respectively. EDS measurement implies the atom percent of Ag is 67.2% and S is 32.8% in matrix that agrees well with the standard stoichiometric composition of Ag2S, as Fig. 3(b) shows.
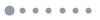
Figure 2.Ag2S ingot prepared by zone melting method
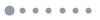
Figure 3.XRD pattern (a) and EDS map (b) of Ag2S
During SEM testing, it is interesting that some micro size particles oozed from the material. Fig. 4(a) shows the original Ag2S surface under 25 kV voltage. However, in a very short time, numerous white particles came up and then gradually grew up for about 30 s, as Fig. 4(b,c) demonstrating. Thereafter, the particle sizes are kept stable. EDS analysis reveals the particle composition is 100% Ag. This result is mainly attributed to the special liquid-like character of Ag2S. As previous literature[15] reported, Ag ions are weakly bonded to the neighbour atoms in silver chalcogenides Ag2M (M=S, Se, Te) semiconductors, and apt to migrate from one site to another if there is sufficient energy force on them. For example, the external heat or voltage are both able to drive Ag ions movement. Therefore, it is easy to understand the high energy electron beam in SEM system plays a significant role causing the deposition of Ag. In fact, similar metal element deposition is also noticed in other type of liquid-like materials, such as Cu2Se, Cu2S, Ag8SnSe6 and so on[16,17,18].
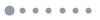
Figure 4.SEM images of original Ag2S surface (a), Ag particles on Ag2S matrix (b) and enlarged morphology of the surface (c)
As for thermoelectric property evaluation, sample 1# for electrical transport measurement is cut parallel to Ag2S solidification direction, and sample 2# for thermal transport testing is processed along perpendicular orientation, as the insert in Fig. 5(a) shows. Here, we should note that such sample processing modes are widely adopted in other zone melting thermoelectric materials, such as Bi2Te3, SnSe, etc[19,20]. In Fig. 5(a), the relationship of temperature with Seebeck coefficient S is displayed. It is found that negative S that means Ag2S is a n-type semiconductor. This conductive behavior might be due to the Ag interstitial ions in crystal structure that act as donor impurities providing additional electrons[14]. Near room temperature, S is about -1200 µV·K-1. As the temperature increased to 440 K, S linearly deceased to -680 µV·K-1. However, when temperature continuously increased to 450 K, S undergoes a sharp decline and the value is around -100 µV·K-1. This dramatic change is mainly attributed to the phase transition of Ag2S. Below 450 K, the material has an α-Ag2S monoclinic structure. Nevertheless, it would transfer to β-Ag2S body centered cubic structure as temperature surpasses 450 K. After that, S maintains a relative stable state regardless the increasing of temperature to 650 K. Fig. 5(b) shows the dependence of conductivity σ on temperature. It is amazing that the σ of α-Ag2S is almost zero before 450 K. However, the σ value sharply jumps to ~40000.5 S·m-1 as the material just finishes phase transition. Then, σ gradually deceases to 33256.2 S·m-1 near 650 K. Fig. 5(c) exhibits power factor PF vs temperature that calculated from PF= S2σ. It is observed that the PF of α-Ag2S is much poor because of its weak conductive property. As for β-Ag2S, PF is practically a constant ~6 µW·cm-1·K-2 in temperature range of 450-650 K.
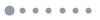
Figure 5.Relationship of Seebeck (a), electrical conductivity σ (b) and power factor PF (c) with temperature
In order to better understand the electrical transport behavior of Ag2S, the Hall properties are also characterized. Fig. 6(a) shows the temperature dependence of carrier concentration nH. Near room temperature, the nH value is on level of ~1017 cm-3. Then, as temperature is increased to the threshold of phase transition, nH is climbed to ~1018 cm-3. This phenomenon is mainly due to the increase of carrier concentration from valence band to conduction band when temperature is added. As expected, when Ag2S is transformed from monoclinic to body centered cubic structure, nH is increased suddenly to ~1019 cm-3 near 450 K. Later, a growing number of carriers are generated in β-Ag2S, and nH rises to a highest ~1020 cm-3 level at 650 K. Fig. 6(b) is the carrier mobility µH diagram varied with temperature. Similar to carrier concentration, µH has a dramatic jump during phase transition. Besides, it is noticed µH is always declined when it is in α-Ag2S and β-Ag2S states, respectively. The maximum µH= 161.6 cm2·V-1·s-1 happens at the moment as Ag2S finishes phase transition.
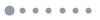
Figure 6.Carrier concentration nH (a) and mobility µH (b) vs temperature
As for thermal transport property, the relationship of total thermal conductivity κ with temperature is given in Fig. 7. When Ag2S is in monoclinic structure, κ is 0.20 W·m-1·K-1 at room temperature and is 0.21 W·m-1·K-1 near 400 K. Here it is necessary to mention that the thermal conductivity is deduced from the measured thermal diffusion coefficient and then approximately calculated through Dulong-Petit law. Thus, there may be certain errors to the accurate κ of the material. However, the result indicates that the thermal conductivity of α-Ag2S is indeed ultralow and very stable. In α-Ag2S, 2 S atoms and 6 Ag atoms form weak chemical bonds along (100) plane. Thus, α-Ag2S would show low phonon vibration frequency because of the weak binding force of S to Ag[11]. As a result, the low-frequency optical branch dominated by Ag atoms can strongly scatter lattice phonons which have similar frequency. This is the key reason why α-Ag2S has ultra-low thermal conductivity. When α-Ag2S turns to β-Ag2S, κ is quickly increased and keeps steady between 450-600 K and the value is ~0.45 W·m-1·K-1. It should be noted that the sulfur element might have slight volatilization during experiment. However, the effect of possible sulfur loss on thermoelectric properties is negligible, as the Ag2S hardly allows stoichiometric deviation of 2∶1 according to the Ag-S phase diagram. Even though any sulfur loss takes place, the excessive Ag would precipitate on the sample surface to maintain Ag2S composition stability.
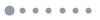
Figure 7.Relationship between κ and temperature
Ultimately, the temperature dependence of ZT is displayed in Fig. 8. Due to the extremely weak electrical transport property, α-Ag2S has very small ZT although its thermal transport is quite low. Nevertheless, the ZT of β-Ag2S is about 0.35 at 450 K and reaches 0.57 near 600 K. The present maximum ZT is comparable to that of Ag2S fabricated by melting method (ZT=0.55, 580 K)[14], and is on the same level compared with other Ag-based materials, such as Ag2Se, Ag2Te, CuAgSe and so on[21,22,23]. This result verifies that such metal sulfide Ag2S is a potential low-temperature thermoeletric material. In the future, Ag2S with element doping is suggested to do help for thermoelectric property improvement.
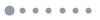
Figure 8.Dependence of ZT with temperature
3 Conclusions
A ϕ18 mm×50 mm Ag2S ingot was fabricated using zone melting method. It undergoes a phase transition from α-Ag2S monoclinic P21/c space group to β-Ag2S body centered cubic structure near 450 K, which has remark influence on its electrical and thermal properties. Ag2S is a n-type semiconductor as the Seebeck constant S is always negative. The PF of α-Ag2S is much poor because of the weak conductive behavior, but the value would suddenly jump to ~6 µW·cm-1·K-2 when phase transition happens. The κ of α-Ag2S and β-Ag2S are ~0.20 W·m-1·K-1 and ~0.45 W·m-1·K-1, respectively. Finally, Ag2S displays a largest ZT= 0.57 near 580 K that means it might be a potential middle-temperature TE material.
References
[1] A AlZAHRANI A, Z ZAINAL, A TALIB Z et al. Study the effect of the heat treatment on the photoelectrochemical performance of binary heterostructured photoanode Ag2S/ZnO nanorod arrays in photoelectrochemical cells. Materials Science Forum, 1002, 187-199(2020). https://www.scientific.net/MSF.1002
[2] S ALHARTHI S, A ALZAHRANI, M A N RAZVI et al. Spectroscopic and electrical properties of Ag2S/PVA nanocomposite films for visible-light optoelectronic devices. Journal of Inorganic and Organometallic Polymers and Materials, 30, 3878-3885(2020). https://doi.org/10.1007/s10904-020-01519-4
[3] Y XIE, H YOO S, C CHEN et al. Ag2S quantum dots-sensitized TiO2 nanotube array photoelectrodes. Materials Science and Engineering B, 177, 106-111(2012). https://linkinghub.elsevier.com/retrieve/pii/S0921510711004193
[4] S KONDRATENKO T, S SMIRNOV M, V OVCHINNIKOV O et al. Nonlinear optical properties of hybrid associates of Ag2S quantum dots with erythrosine molecules. Optik-International Journal for Light and Electron Optics, 200, 163391(2020). https://linkinghub.elsevier.com/retrieve/pii/S0030402619312896
[5] C YOU J, B ZHAN S, J WEN et al. Construction of heterojunction of Ag2S modified yttrium manganate visible photocatalyst and study on photocatalytic mechanism. Optik-International Journal for Light and Electron Optics, 217, 164900(2020). https://linkinghub.elsevier.com/retrieve/pii/S0030402620307361
[6] R VOGEL, P HOYER, H WELLER. Quantum-sized PbS, CdS, Ag2S, Sb2S3, and Bi2S3 particles as sensitizers for various nanoporous wide-bandgap semiconductors. The Journal of Physical Chemistry, 98, 3183-3188(1994). https://pubs.acs.org/doi/abs/10.1021/j100063a022
[7] M DONG Z, S SUN H, J XU et al. Preparation of macroscopical long Ag2S nanowire clusters characteristics. Acta Physica Sinica, 60, 676-680(2011).
[8] P DU Y, B XU, T FU et al. Near-infrared photoluminescent Ag2S quantum dots from a single source precursor. Journal of the American Chemical Society, 132, 1470-1471(2010). https://pubs.acs.org/doi/10.1021/ja909490r
[9] Y ZHANG, S HONG G, J ZHANG Y et al. Ag2S quantum dot: a bright and biocompatible fluorescent nanoprobe in the second near-infrared window. ACS Nano, 6, 3695-3702(2012). https://pubs.acs.org/doi/10.1021/nn301218z
[10] I HWANG, M SEOL, H Kim et al. Improvement of photocurrent generation of Ag2S sensitized solar cell through co-sensitization with CdS. Applied Physics Letters, 103(2013).
[11] X SHI, Y CHEN H, F HAO et al. Room-temperature ductile inorganic semiconductor. Nature Materials, 17, 421-426(2018). https://doi.org/10.1038/s41563-018-0047-z
[12] W CHEN Z, Y ZHANG X, Q LIN S et al. Rationalizing phonon dispersion for lattice thermal conductivity of solids. National Science Review, 5, 888-894(2018). https://academic.oup.com/nsr/article/5/6/888/5104387
[13] G JEFFREY S, T AGNE M, G RAMYA. Thermal conductivity of complex materials. National Science Review, 6, 380-381(2019). https://academic.oup.com/nsr/article/6/3/380/5423892
[14] T WANG, Y CHEN H, F QIU P et al. Thermoelectric properties of Ag2S superionic conductor with intrinsically low lattice thermal conductivity. Acta Physica Sinica, 68, 18-26(2019).
[15] Y CHEN H, M YUE Z, D REN D et al. Thermal conductivity during phase transitions. Advanced Materials, 31, 1806518(2019).
[16] P LU, L LIU H, X YUAN et al. Multiformity and fluctuation of Cu ordering in Cu2Se thermoelectric materials. Journal of Materials Chemistry A, 3, 6901-6908(2015). http://xlink.rsc.org/?DOI=C4TA07100J
[17] B ZHANG Y, W WANG Y, L XI L et al. Electronic structure of antifluorite Cu2X (X=S, Se, Te) within the modified Becke- Johnson potential plus an on-site Coulomb U. Journal of Chemical Physics, 140, 074702(2014). http://aip.scitation.org/doi/10.1063/1.4865257
[18] M JIN, Q LIN S, W LI et al. Fabrication and thermoelectric properties of single-crystal argyrodite Ag8SnSe6. Chemistry of Materials, 31, 2603-2610(2019). https://pubs.acs.org/doi/10.1021/acs.chemmater.9b00393
[19] J JIANG, D CHEN L, Q BAI S et al. Thermoelectric properties of p-type (Bi2Te3)x(Sb2Te3)1-x crystals prepared via zone melting. Journal of Crystal Growth, 277, 258-263(2005). https://linkinghub.elsevier.com/retrieve/pii/S0022024804021335
[20] X WANG, T XU J, Q LIU G et al. Texturing degree boosts thermoelectric performance of silver-doped polycrystalline SnSe. NPG Asia Materials, 9, 426(2017).
[21] B WANG X, F QIU P, S ZHANG T et al. Compound defects and thermoelectric properties in ternary CuAgSe-based materials. Journal of Materials Chemistry A, 3, 13662-13670(2015). http://xlink.rsc.org/?DOI=C5TA02721G
[22] T DAY, F DRYMIOTIS, S ZHANG T et al. Evaluating the potential for high thermoelectric efficiency of silver selenide. Journal of Materials Chemistry C, 1, 7568-7573(2013). http://xlink.rsc.org/?DOI=c3tc31810a
[23] Z PEI Y, A HEINZ N, J SNYDER G. Alloying to increase the band gap for improving thermoelectric properties of Ag2Te. Journal of Materials Chemistry, 21, 18256-18260(2011). http://xlink.rsc.org/?DOI=c1jm13888j