I. V. Aleksandrova, E. R. Koresheva, "Advanced fuel layering in line-moving, high-gain direct-drive cryogenic targets," High Power Laser Sci. Eng. 7, 03000e38 (2019)

Search by keywords or author
- High Power Laser Science and Engineering
- Vol. 7, Issue 3, 03000e38 (2019)
Abstract
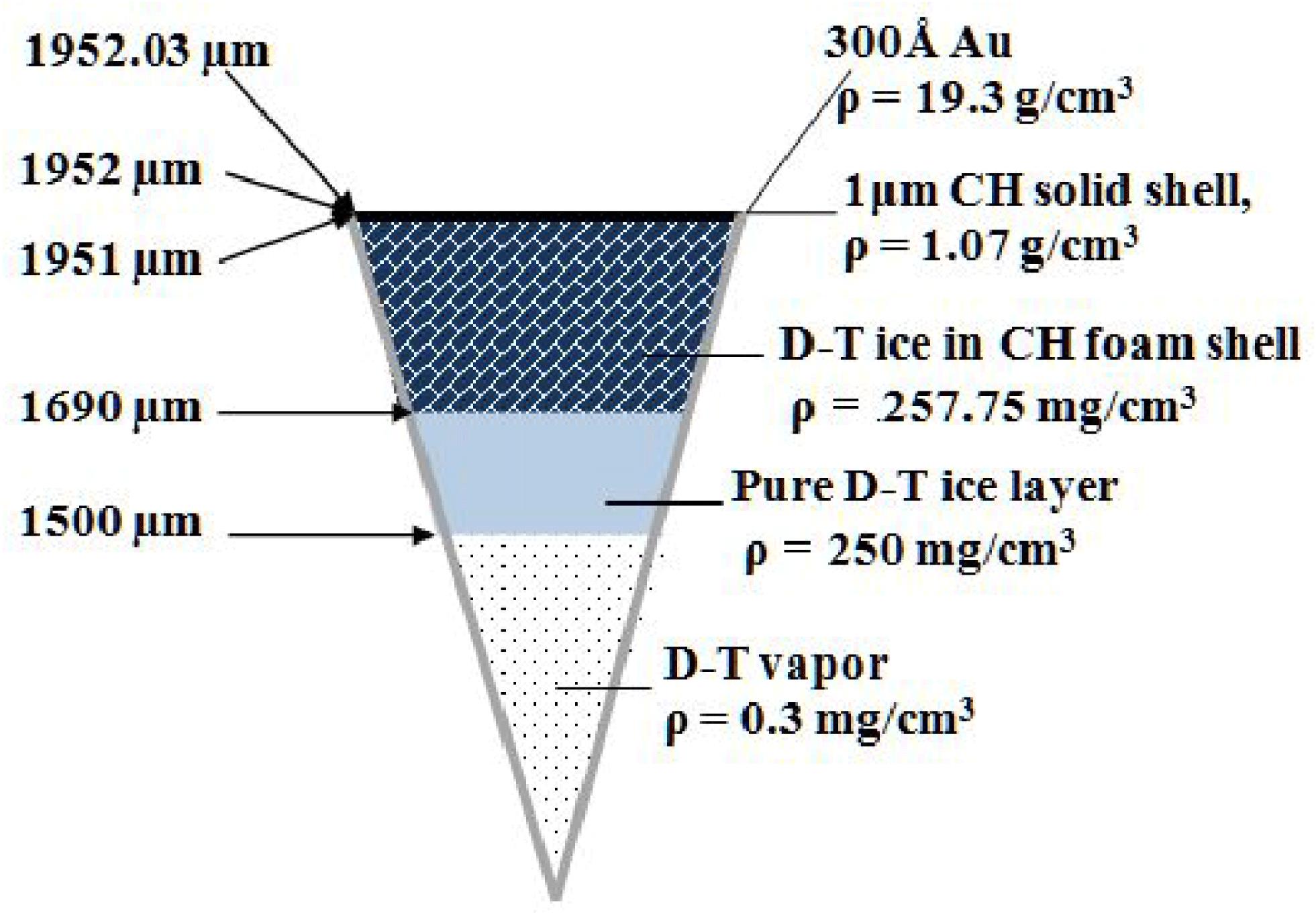
Set citation alerts for the article
Please enter your email address