Author Affiliations
University of California, Department of Electrical and Computer Engineering, San Diego, La Jolla, California, United Statesshow less
Fig. 1. Schematic presentation of TLD film deformation forming optical liquid lattices (blue) due to surface tension effects triggered by interference of surface optical modes (red). (a) 2-D plasmonic liquid lattice formed by interference of SPPs. (b), (c) Suspended and supported photonic liquid lattice, respectively, formed by interference of photonic slab WG modes. Gain can be introduced into the suspended structure (c) either to the liquid or to the dielectric supporting membrane. The lateral dimensions of the liquid slots, which are bounded by solid dielectric walls (not shown) are and . (d)–(f) The corresponding 1-D optical liquid lattices in a liquid slot of length induced by pairs of (d) counterpropagating SPPs or (e) and (f) slab WG modes.
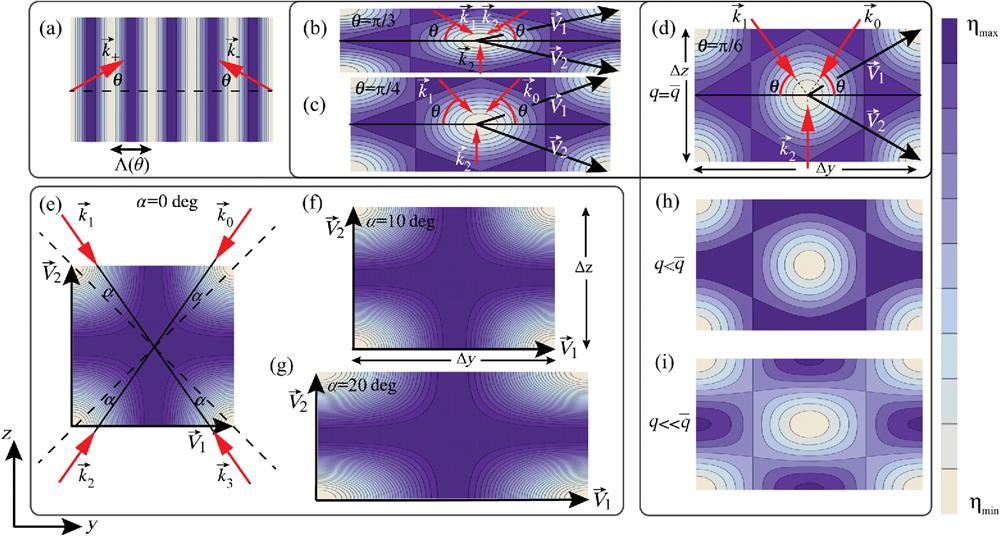
Fig. 2. Optical liquid lattices formed from a TLD film due to interference of SPPs or WG plane wave modes with propagating direction marked with red arrows. (a) One-dimensional lattice formed by interference of two surface optical plane waves with -dependent periodicity given by Eq. (6). (b)–(d) Two-dimensional lattices of hexagonal symmetry and (e)–(g) rectangular symmetry, where and are the corresponding primitive vectors. The symmetry of the liquid lattice and can be controlled by the relative angles of the interfering beams; (b) and (c) are lattices with hexagonal broken/distorted symmetry, whereas (d) is hexagonal symmetric lattice; (e) (square symmetry), (f) , and (g) correspond to rectangular symmetry. (h), (i) Optical liquid lattice for different values of which results in (d) a phase transition from a hexagonal lattice to (h) merging of the triangular sites and (i) face-centered cubic lattice. The 2-D liquid lattices are normalized by and defined in Eq. (9) and below Eq. (11).
Fig. 3. Numerical simulation results presenting the bandstructure of photonic slab WG modes (i.e., frequency as a function of the corresponding directions in the k-space) formed in a suspended photonic liquid lattice of hexagonal symmetry and hexagonal broken symmetry. (a)–(c) Bandstructure of TE slab WG modes in a suspended photonic liquid lattice presented in Figs. 1(b)–1(d) due to interference formed by three plane waves at angles (a) , (b) , and (c) . (d)–(f) Bandstructure of TM slab WG modes at interference angles (d) , (e) , and (f) . Two Dirac points emerge at the angle at frequencies around 353 and 349 THz, respectively, marked at (c) and (f) by black arrows. In the simulation, the refractive index is 1.409, the mean TLD film thickness is 450 nm, and the peak-to-peak undulation amplitude is 300 nm.
Fig. 4. Numerical simulation results presenting lasing frequencies tuning in plasmonic liquid lattice and suspended photonic liquid lattice, of hexagonal and rectangular symmetries, respectively, where the angles of the interfering “writing beams” and are described in Fig. 2. (a) Lasing frequencies tuning of WG TE and TM modes for hexagonal symmetric photonic liquid lattice with values , 48 deg, 51 deg, 54 deg, 57 deg, and 60 deg (in radians) labeled as 1 to 6 near the blue and red disks, respectively; SPP modes in rectangular symmetric plasmonic liquid lattice with values , 5 deg, 10 deg, 15 deg, and 20 deg, labeled as 1 to 5 near the red squares, respectively. (b) The location of the corresponding lasing modes in the reduced Brillouin zone in the k-space.