Jingxuan Zhang, Chenni Xu, Patrick Sebbah, Li-Gang Wang, "Diffraction limit of light in curved space," Photonics Res. 12, 235 (2024)

Search by keywords or author
- Photonics Research
- Vol. 12, Issue 2, 235 (2024)
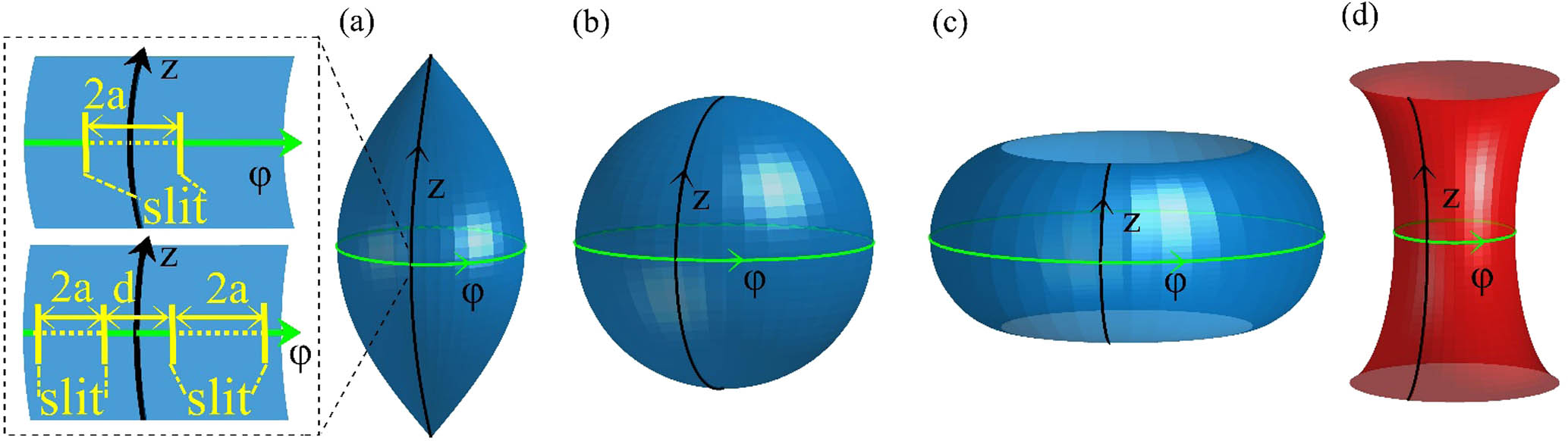
Fig. 1. SORs with constant (a)–(c) positive and (d) negative Gaussian curvature K . Here (a) spindle with r 0 < R , (b) sphere with r 0 = R , and (c) bulge with r 0 > R , where R = | K | − 1 2 is the radius of Gaussian curvature, and r 0 is an initial rotational radius (or radius of equator) at z = 0 . The black/green solid lines are the lines of longitude/equator. The inset on the left side of (a) shows the schematic of a single slit (up) and double slits (down) on surface, which are located at the equator (z = 0 ).
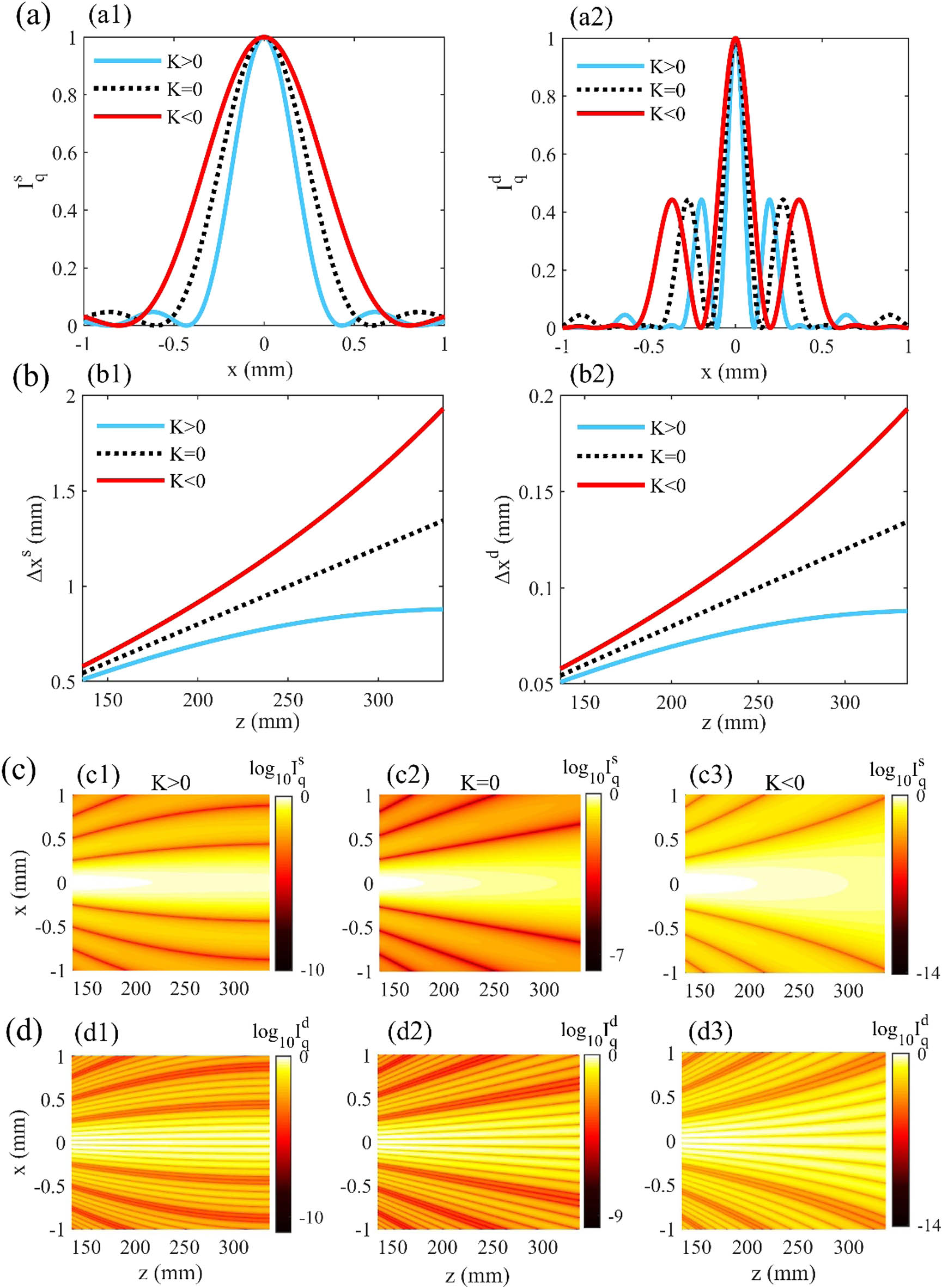
Fig. 2. Diffraction and interference on curved space. (a) Intensity distributions of Fraunhofer (a1) single-slit diffraction and (a2) double-slit interference of light at z = 300 mm on SORs with different Gaussian curvature. (b) Variations of the central fringe widths Δ x s and Δ x d for (b1) single-slit diffraction and (b2) double-slit interference with the propagation distance in different SORs. (c), (d) Evolutions of light fields of Fraunhofer (c) single-slit diffraction and (d) double-slit interference in different spaces: (c1), (d1) K > 0 , (c2), (d2) K = 0 , and (c3), (d3) K < 0 . Here the parameters for SORs with R = 220 mm are taken as K = 20.66 m − 2 for K > 0 , and K = − 20.66 m − 2 for K < 0 . Other parameters are λ = 400 nm , r 0 = 100 mm , a = 0.1 mm , d = 0.2 mm in (a2) and d = 0.8 mm in (d1)–(d3) for better visualization.
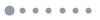
Fig. 3. Effect of Gaussian curvature K of SORs on the change of the diffraction limit at different propagation distance z . The black dashed line denotes the case for the diffraction limit in flat space. The zero value of δ means no effect on diffraction limit, compared with the case in flat space.
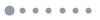
Fig. 4. Diffraction of light fields along different propagation directions on (a) spindle, (b) sphere, and (c) hyperboloid. The propagation direction is described by the angle Θ between the longitude (black solid lines) and the incident direction. Here three typical propagation directions with Θ = 30 ° , 45°, and 60° are considered, and the corresponding geodesics are indicated by the white, yellow, and green curves. D is the propagation distance along these geodesics. The initial central position of the input plane is z i = − 230.384 mm , − 295.134 mm , and − 166.415 mm in (a)–(c), respectively, the parameters of these SORs are r 0 = 100 mm , 220 mm, and 100 mm in (a)–(c), respectively, and other parameters are the same as in Fig. 2 .
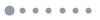
Fig. 5. Diffraction of light fields along different propagation directions on (a) an FP surface, (b) a SdS 2 surface, and (c) a PPSS surface. The propagation direction Θ is defined the same as in Fig. 4 , and three typical directions Θ = 3 ° , 9°, and 15°, corresponding to the white, yellow, and green geodesics, respectively, are plotted. The surfaces are colored by blue/red to indicate the regions with positive/negative Gaussian curvature. The black dotted lines in (a3), (b3), and (c3) show the variation of diffraction limit in flat space. Here the initial central positions of the input plane are r i = 460 mm in (a), r i = 283.66 mm in (b), and z i = 100 mm in (c), the Schwarzschild radius r s = 30 mm in (a) and (b), and the cosmological constant is Λ = 33.33 m − 2 in (b). In (c), we take α = 100 mm , B = 10 mm , β = 20 mm , and ε = − 1.25 π . Other parameters are the same as in Fig. 2 .
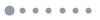
Fig. 6. Dependence of the relative quantity δ on the propagation direction at different propagation distances (a) D = 400 mm and (b) D = 500 mm . Other parameters are the same as in Figs. 4 and 5 .
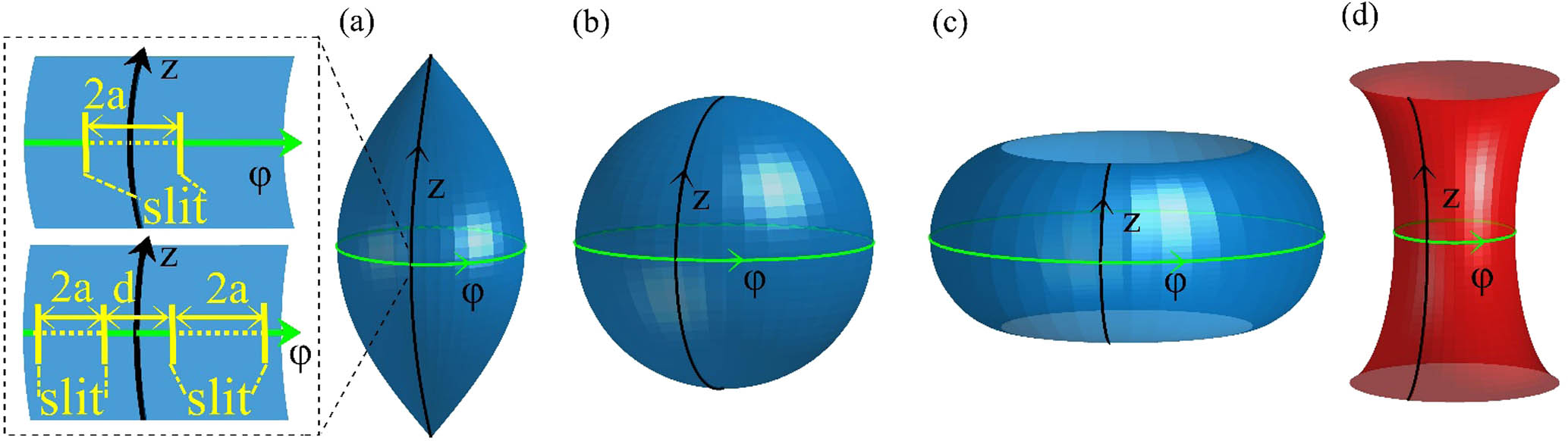
Set citation alerts for the article
Please enter your email address