Andrey Alekseevich Ionin, Igor Olegovich Kinyaevskiy, Yuri Mikhailovich Klimachev, Andrey Yurievich Kozlov..., Darya Ivanovna Kormashova*, Andrey Alexandrovich Kotkov, Yury Alekseevich Mityagin, Sergey Alexandrovich Savinov, Adilya Maratovna Sagitova, Dmitry Vasilievich Sinitsyn and Maxim Vladimirovich Ionin|Show fewer author(s)
Abstract
Terahertz (THz) NH3 lasing with optical pumping by electron-beam-sustained discharge “long” () CO2 laser pulses was obtained. The NH3 laser emission pulses and the “long” pulses of the CO2 pump laser were simultaneously measured with nanosecond response time. The NH3 lasing duration and its delay with respect to the pump pulse were measured for various CO2 laser pulse energies. For the CO2 laser pump line 9R(30), three wavelengths of 67.2, 83.8, and 88.9 µm were recorded. For the CO2 laser pump line 9R(16), only a single NH3 laser line with a wavelength of 90.4 µm was detected.1. Introduction
Currently, terahertz (THz) radiation is already actively used in industry[1], medicine[2], the study of cultural heritage sites[3], and other fields. But, for solving some special problems (for example, plasma diagnostics[4], remote detection of explosives behind obstacles[5]), it is of interest to develop high-power sources of laser radiation with a special spectral composition in the THz range. One of the most spectroscopically studied gas lasers of this type is a laser. THz emission on transitions can be obtained by optical pumping by a laser, which is an efficient and technically well-developed method. The first studies, to the best of our knowledge, with the THz laser were aimed at studying its spectral capabilities. Therefore, a high-power pulsed transversely excited atmosphere (TEA) laser for optical pumping and an optical cell with THz cavities of very low output ratio, with input and output radiation through millimeter holes, were used (see, for example, Refs. [6,7]). This made it possible to obtain lasing on dozens of transitions in the wavelength range from to . Later studies added new laser lines to the set, the total number of which approaches 100[8]. In particular, it was found that for the laser pump line 9R(16), the laser can produce emission on up to 10 wavelengths of the THz range, for the 9R(30) pump line, more than 10 wavelengths, and using other pump lines led to laser lines.
Several research groups studied in detail lasing with a wavelength of for the laser pump line 10P(32) and for the 9R(16) pump line[9–12]. Such an interest in these wavelengths of the THz laser is apparently associated, firstly, with the high efficiency of emission conversion (1.6% for [10] and 1.7% for [9]), and, secondly, with the prospect of using such THz emission for plasma diagnostics in electrodynamic accelerators and tokamaks with a strong magnetic field[13]. Therefore, for these wavelengths, in contrast to others, the shape of a THz laser pulse was studied in comparison with a pump pulse. In Ref. [10], the shape of a single-line pulse with a wavelength of was the same as for a laser pulse of duration. At the same time, a lasing pulse with a wavelength of started only after the first, most powerful peak of the pump pulse and replicated the shape of a low-energy “tail” of a laser pulse[12].
In Ref. [11], laser cavity -switching was implemented for THz radiation. Peak power at a wavelength of 152 µm approached 10 kW at the pulse duration of . The authors studied optimal -switching time relative to a laser pulse starting point, which, as the author noted, had an “amazing” delay of 1.5–2.0 µs, i.e., an order of magnitude longer than the pump pulse duration itself.
Sign up for Chinese Optics Letters TOC. Get the latest issue of Chinese Optics Letters delivered right to you!Sign up now
Two research groups were engaged in solving the problem of obtaining high power in the atmospheric transparency window near a wavelength of [14,15] with optical pumping by the 10R(14) TEA laser line. Along with the emission on the line, there was more powerful emission at wavelengths of 257 and 281 µm. It was shown[14] that laser pulses with a duration up to are necessary for optimal lasing on the 2143 µm line, which started approximately 400 ns after the pump pulse beginning and continued until its end. In Ref. [15], output energy at the obtained wavelengths for a pump pulse with energy of 4 J was measured: 7 mJ at 257 µm, 2 mJ at 281 µm, and 0.4 mJ at 2143 µm. The energy efficiency for the 2143 µm line and for the 257 µm line was and 0.2%, respectively. However, the authors did not study the temporal shape of the laser pulse with a wavelength of 281 µm belonging to the same laser cascade as a pulse with a 2143 µm wavelength.
Thus, it was demonstrated that an optically pumped laser can operate on a large number of THz lines with relatively high efficiency, including lines suitable for plasma diagnostics. However, it should be noted that up to now the cascade mechanism of THz laser operation optically pumped by a laser has not been studied in detail. THz laser pulse temporal shapes were mainly detected under experimental conditions, when lasing took place at a single wavelength[9–12], or the pulse shape was only studied for a single one out of a set of wavelengths[14,15].
For plasma diagnostics, it is of great interest to apply a laser operating at several wavelengths simultaneously, which makes it possible to abandon the optical scheme for combining laser beams of different lasers traditionally used in such experiments (see, for example, Ref. [4]). To arrange multifrequency THz lasing, it makes sense to study characteristics of the laser pumped by laser pulses with a duration that is orders of magnitude longer than the molecule vibrational relaxation time ([13]), i.e., an implementation of quasi-CW optical pumping.
The objective of our research was just the implementation of such an optical pumping of a laser by a “long” pulse laser with pulse duration up to and studying their temporal characteristics with a nanosecond resolution under concurrent operation of and lasers. As a pump laser, an electron-beam-sustained discharge (EBSD) laser was applied. Due to the use of such laser pulses, the probability of obtaining multifrequency THz lasing quite increases. First, due to intermode beats, they have a spiked structure with a high () peak power an order of magnitude close to the peak power of short pulses used in Refs. [6,7]. Second, in contrast to the above mentioned papers, they have a pump pulse energy that is 2–3 orders of magnitude higher due to the pulse duration.
2. Methods
We used an optical pumping scheme similar to that applied in Ref. [7]. The optical scheme of our experiment is shown in Fig. 1.
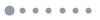
Figure 1.Optical scheme of the experiment: 1, 5, CO2 laser rear mirrors; 2, EBSD CO2 laser chamber; 3, 7, 9, plane-parallel BaF2 plates; 4, plane-parallel ZnSe plate; 6, diffraction grating; 8, 22, spherical mirrors; 10, photodetector; 11, power and energy meter; 12, 21, flat mirrors; 13, lens; 14, gas cell input window; 15, gas input channel; 16, output to pressure meter; 17, mirrors of THz cavity; 18, Mylar film; 19, parabolic mirror; 20, quartz plate; 23, detector of THz radiation.
In our experiment, we applied an EBSD laser with the gas mixture under gas pressure of 0.30 atm (1 atm = 1.013 × 105 Pa). Plane reflective mirror 1 was installed directly on the EBSD laser chamber 2. Plane-parallel plate 3 made of was used as a Brewster window. The optical cavity of the laser consisted of rear reflective mirror 1, plane-parallel ZnSe plate 4, and diffraction grating 6 (100 grooves/mm). Diffraction grating 6 provided lasing at a specific vibrational–rotational transition. To increase the output energy, an additional rear reflective mirror 5 was used. According to our estimates, the radiation output ratio when using plane-parallel plate 4 was . Plane-parallel plates 7 and 9 split the laser beam, directing part of it to power and energy meter 11 (OPHIR 3A-SH) and photodetector 10 (PEM-L-3, response time 0.5 ns). Spherical mirror 8 (radius of curvature 100 cm) was used for focusing the laser beam. The rest of the laser beam () was directed by plane mirror 12 and focusing lens 13 (focal length 9 cm) into a gas cell with ammonia.
A gas cell 20 cm long was bounded by NaCl optical window 14 and 18 of 0.1 mm thickness made of Mylar. Inside the gas cell, two plane brass mirrors 17 located at a distance of 12 cm from each other had a central hole of 2 mm in diameter, through which the pump laser beam came into the cell, and THz emission was extracted. Gaseous ammonia came into the cell through port 15. Gas pressure was controlled through port 16 with a Busch capsule vacuum gauge (0–25 mbar).
The THz laser beam from the gas cell was directed into detector 23 (RS 0.4–4 T bolometer, manufactured by LLC SKONTEL, response time ) by parabolic mirror 19 (focal length 15 cm), flat mirror 21, and spherical mirror 22 (radius of curvature 50 cm). The laser and emissions in the mid-IR were cut off by crystalline quartz plate 20.
3. Experiment
Our experiments were carried out with the laser operating on spectral lines, for which the largest number of laser lines was obtained[7,16], namely, 9R(30) () and 9R(16) () corresponding to [G − ν2; sR(5, 0)] and [G − ν2; aR(6, 0)] absorbing transitions of ammonia molecules. The duration of the pump pulses reached , and the energy of the pump pulses was up to . The optimal gas pressure in the cell with ammonia for lines 9R(16) and 9R(30) was 8 mbar and 2 mbar, respectively. An increase or decrease of the pressure of relative to the optimal value led to a decrease in the THz radiation energy. In optically pumped gas lasers, the lasing directly depends on the absorbed pump radiation. The emission on the 9R(16) laser line is less absorbed by molecules as compared to the emission on the 9R(30) line for two reasons. Firstly, due to different absorption lines of the transition, the 9R(30) line emission is absorbed by molecules from the sR(5, 0) level, while the 9R(16) line emission is absorbed from a higher aR(6, 0) level. Secondly, the 9R(30) line is off the absorption line center, and the 9R(16) line is off[16]. With an increase in pressure up to 2 mbar , the absorption of radiation on both these lines and, as a result, THz lasing increases simply due to an increase in the number of absorbing (emitting) particles. However, with a subsequent increase in gas pressure, the broadening of the absorption lines grows up. In the case of the 9R(30) line, this broadening reduces the absorption, and, in the case of the 9R(16) line, the broadening gives an additional factor to the absorption enhancement. Therefore, the optimal ammonia pressure for THz lasing pumped by the 9R(16) line is 8 mbar. A subsequent increase in the pressure reduces the lasing due to the third factor—the increase in the rotational relaxation of the excited molecules.
There was a certain intermediate gas pressure (4.5 mbar), at which THz radiation was observed when pumped by both lines of the laser.
Some examples of the laser and laser pulses are shown in Figs. 2 and 3 (ammonia pressure 4.5 mbar). The insets show initial sections of the pulses. The temporal shape of the laser pulses is calibrated to the measured values of the pulse energy . The time instant corresponds to the beginning of the EBSD pump pulse, the duration of which is . THz lasing was observed during the most powerful part of the laser pulse, when the peak power exceeded for the 9R(16) pump line and for the 9R(30) pump line. Low-frequency power fluctuations () in the laser pulse were replicated in the temporal form of the laser pulse (see inset to Fig. 2). At the same time, high-frequency power oscillations () in a “long” laser pulse associated with intermode beating[17] were not always replicated in the temporal shape of the laser pulse (they are absent in Fig. 2, but they are in Fig. 3). Apparently, this is due to differences in the capabilities of the measuring equipment: the IR detector had two times better response time (0.5 ns) and times better signal-to-noise ratio than the THz one.
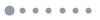
Figure 2.Pulses of CO2 laser (bottom) and NH3 laser (top) pumped by the line 9R(16). Epulse = 0.59 J.
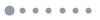
Figure 3.Pulses of CO2 laser (bottom) and NH3 laser (top) pumped by the line 9R(30). Epulse = 0.91 J.
It can be seen from Fig. 2 that the shape of the laser pulse replicates the shape of the most powerful part of the pump pulse. However, in Fig. 3, we observe two pronounced maxima in the THz laser pulse at sufficiently stable laser output power.
We assume that this is a manifestation of the cascade mechanism of lasing explaining multifrequency lasing when the laser operating on this particular line is used for pumping, which was observed, in particular, in Refs. [6,16]. This was confirmed by subsequent measurements of the laser spectrum.
The instability of the THz pulse train is explained by the unstable pump laser pulse train. Therefore, it is possible to analyze only both THz pulse time delays relative to the pump pulse and the laser pulse duration.
Figure 4 demonstrates dependences of lasing time delay relative to the pump pulse and the duration of laser pulses on the laser pulse energy for the pump lines 9R(16) and 9R(30).
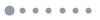
Figure 4.Dependence of the NH3 laser pulse duration τpulse and the delay time τdelay on the CO2 laser pulse energy for the pump lines 9R(16) and 9R(30).
It can be seen from Fig. 4 that at the same pump energies, the duration of laser pulses is longer for the 9R(30) pump line than for the 9R(16) one. In both cases, the delay of the lasing onset goes down, and the laser pulse duration goes up with the laser pulse energy increase. The time delay of lasing onset is for the 9R(16) line and for the 9R(30) line. The duration of the laser pulse depending on the laser pulse energy ranged from 10 to 25 µs and from 25 to 40 µs for the 9R(16) and the 9R(30) lines, respectively. The smaller delay in the onset of lasing under pumping by the 9R(30) line as compared to the one by the 9R(16) line can be explained by the difference in the laser radiation absorption. At the pressure of 4.5 mbar, apparently, the absorption of the 9R(30) line is still stronger than the absorption of the 9R(16) line. Therefore, the threshold value of the laser gain is reached faster when pumped by the 9R(30) line than when pumped by the 9R(16) line.
To measure the wavelength of THz output, a diffraction grating of 6 grooves/mm with a blaze angle of 12° was used. It was installed instead of flat mirror 21 (see Fig. 1). In this series of experiments, THz detector 23 instead of LLC SKONTEL pyroelectric detector of LLC INFRATEKH-P with a response time of was used. Based on the THz beam diameter (12.6 mm diameter on level), the spectral resolution was in the region of 60 µm and in the region of 100 µm. (The accuracy of the diffraction grating angle of rotation was 0.02°, which corresponded to an accuracy of 0.1 µm.) The spectrum of THz emission was measured, first of all, for the pump 9R(30) laser line, because, in this case, we observed an unusual form of THz lasing (see Fig. 3). The resulting spectrum is shown in Fig. 5.
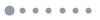
Figure 5.Spectrum of THz emission obtained for the pump CO2 laser line 9R(30); ammonia pressure 2.5 mbar.
Three lines of THz emission from were recorded with wavelengths of , , and . The strongest of them was the line with a wavelength of 83.8 mm. Identification (ID) of the transitions was carried out according to the results in Ref. [6], the transitions ID is shown in Fig. 5 above the corresponding columns of the diagram. Under these experimental conditions, the main part of THz emission energy was obtained on the so-called “refilling” transition, i.e., transition between the levels of the ground vibrational state. It arises due to the equalization of the populations’ nonequilibrium caused by the pumping of molecules by the laser onto the first excited vibrational state . It should be noted that the wavelengths fixed by us do not exclude lasing on other THz transitions. Firstly, the possibilities of detecting THz radiation can be limited by the spectral sensitivity of the used pyroelectric detector and the characteristics of the reflectivity of the used diffraction grating. Secondly, the spectrum of THz lasing strongly depends on gas pressure in the gas cell. In particular, in our case, all other conditions being equal, an increase in the pressure up to 5.0 mbar resulted in a twofold enhancement in the THz signal at 67.2 and 88.9 µm, while the emission on the 83.8 µm line disappeared. Therefore, in Fig. 3, we could observe the generation of at least two cascade-coupled lines of the first excited vibrational state at wavelengths 67.2 µm (the first half of the lasing pulse) and 88.9 µm (the second half of the lasing pulse).
For the 9R(16) laser pump line, THz lasing was studied at ammonia pressures of 10.0, 5.5, 3.0, and 2.0 mbar. At each gas pressure, four possible lasing lines with wavelengths of , , , and were tested; however, THz emission was detected only at the 90.4 µm line. The ID of transitions was carried out according to the results of Ref. [16]. The generated 90.4 µm line corresponds to transition . The lines on which no THz radiation was detected, 63.3, 72.8, and 67.2 µm, correspond to the transitions , (“refilling” transitions), and , respectively. Figure 6 shows that the energy of laser pulses at a wavelength of 90.4 µm increases with enhanced pressure and is maximal at 10 mbar. In all the cases, the output energy grew up with increasing pump energy.
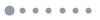
Figure 6.Dependence of the NH3 laser output with a wavelength of 90.4 µm on the CO2 laser pulse energy for the 9R(16) pump line at ammonia pressure of 10.0, 5.5, 3.0, and 2.0 mbar.
More detailed conclusions about the cascade mechanism of THz lasing can be made only in the case of studying the temporal shape with nanosecond resolution at each of the possible laser wavelengths.
4. Conclusion
Thus, in this paper, THz laser pulses and “long” () pump laser pulses were measured simultaneously with nanosecond resolution. The time delay of the lasing onset [ for the 9R(16) line and for the 9R(30) line] went down, and the pulse duration went up with an increase in the laser pulse energy. The duration of the laser pulse, depending on the energy of the laser pulse, ranged from 10 to 25 µs and from 25 to 40 µs for the 9R(16) and 9R(30) lines, respectively.
The wavelengths of THz radiation from the laser were also measured. For the laser pump line 9R(30), these wavelengths were 67.2, 83.8, and 88.9 µm. The wavelength of the strongest line was 83.8 µm. For the laser pump line 9R(16), only the single 90.4 µm spectral line was recorded.
References
[1] M. Naftaly, N. Vieweg, A. Deninger. Industrial applications of terahertz sensing: state of play. Sensors, 19, 4203(2019).
[2] E. P. J. Parrott, Y. Sun, E. Pickwell-MacPherson. Terahertz spectroscopy: its future role in medical diagnoses. J. Mol. Struct., 1006, 66(2011).
[3] K. Fukunaga. Terahertz applications in art conservation. Handbook of Terahertz Technology for Imaging, Sensing and Communications, 615(2013).
[4] Z. Vereshchinski, V. G. Gerasimov, E. P. Gorbunov, A. Kasperchuk, M. Lesnewski, A. Y. Molchanov, M. Padukh, K. Paprotski. Two-wave multichannel laser submillimeter interferometer (TMLSI) for horizontal probing of the plasma in the T-15. Sov. J. Plasma Phys., 18, 106(1992).
[5] J. F. Federici, D. Gary, R. Barat, Z.-H. Michalopoulou. Chapter 11–Detection of explosives by terahertz imaging. Counterterrorist Detection Techniques of Explosives, 323(2007).
[6] K. Gullberg, B. Hartmann, B. Kleman. Submillimeter emission from optically pumped 14NH3. Phys. Scr., 8, 177(1973).
[7] Y. Nishi, Y. Horiuchi, S. Wada, N. Sokabe, A. Murai. New laser emission from NH3 optically-pumped by TE–CO2 laser. Jpn. J. Appl. Phys, 21, 719(1982).
[8] S. Marchetti, R. Simili. New FIR emissions in ammonia by pumping with a line narrowed high pressure CO2 laser. Int. J. Infrared Millim. Waves, 20, 2083(1999).
[9] H. Hirose, S. Kon. Compact, high power FIR NH3 laser pumped in a three mirror CO2 laser cavity. Int. J. Infrared Millim. Waves, 5, 1571(1984).
[10] H. Hirose, S. Kon. Compact, high-power FIR NH3 laser pumped in a CO2 laser cavity. IEEE J. Quantum Electron., 22, 1600(1986).
[11] T. E. Wilson. A high-power far-infrared NH3 laser pumped in a three-mirror CO2 laser cavity with optically-switched cavity-dumping. Int. J. Infrared Millim. Waves, 14, 303(1993).
[12] Z. Jiu, D. Zuo, L. Miao, Z. H. Cheng. An efficient high-energy pulsed NH3 terahertz laser. Int. J. Infrared Millim. Waves, 31, 1422(2010).
[13] V. A. Mishchenko, Y. V. Petrushevich, D. N. Sobolenko, A. N. Starostin. High-power terahertz optically pumped NH3 laser for plasma diagnostics. Plasma Phys. Rep., 38, 460(2012).
[14] P. Woskoboinikow, J. Machuzak, W. Mulligan. A high-power 140 GHz ammonia laser. IEEE J. Quantum Electron., 21, 14(1985).
[15] S. Marchetti, M. Martinelli, R. Simili, R. Fantoni, M. Giorgi. Efficient millimetre far infrared laser emissions in different molecular systems. Infrared Phys. Technol., 41, 197(2000).
[16] T. Yoshida, N. Yamabayashi, K. Miyazaki, K. Fujisawa. Infrared and far-infrared laser emissions from a TE CO2 laser pumped NH3 gas. Opt. Commun., 26, 410(1978).
[17] C. Beairsto, R. Walter, A. A. Ionin, A. A. Kotkov, R. Penny, L. A. Seleznev, S. Squires. Control of laser radiation parameters: high-frequency temporal structure of laser and phase-conjugated signals in intracavity degenerate four-wave mixing of radiation from electron-beam-controlled discharge CO2 and CO lasers in their active media. Quantum Electron., 27, 614(1997).