Yosuke Nagasawa, Akira Hirano, "Review of encapsulation materials for AlGaN-based deep-ultraviolet light-emitting diodes," Photonics Res. 7, B55 (2019)

Search by keywords or author
- Photonics Research
- Vol. 7, Issue 8, B55 (2019)
![Cracks in silicone encapsulation of AlGaN-based (a) 270 nm, (b) 287 nm, and (c) 301 nm LEDs after the reliability tests. The time and the operation current during the reliability tests are indicated at the top of the photographs. The insets are magnified images of the cracks. [Figure 1(c) is taken from Ref. [27]. Copyright Japan Society of Applied Physics.]](/richHtml/prj/2019/7/8/08000B55/img_001.jpg)
Fig. 1. Cracks in silicone encapsulation of AlGaN-based (a) 270 nm, (b) 287 nm, and (c) 301 nm LEDs after the reliability tests. The time and the operation current during the reliability tests are indicated at the top of the photographs. The insets are magnified images of the cracks. [Figure 1(c) is taken from Ref. [27]. Copyright Japan Society of Applied Physics.]
![Absorption spectrum of silicone with side chains of methyl groups with the molecular structure shown as an inset. The weak absorption observed between approximately 250 and 280 nm is considered to be that of the polymerization initiator. (Reproduced from Ref. [27]; copyright Japan Society of Applied Physics.)](/richHtml/prj/2019/7/8/08000B55/img_002.jpg)
Fig. 2. Absorption spectrum of silicone with side chains of methyl groups with the molecular structure shown as an inset. The weak absorption observed between approximately 250 and 280 nm is considered to be that of the polymerization initiator. (Reproduced from Ref. [27]; copyright Japan Society of Applied Physics.)
![(a) Transmittance spectra of p-BVEs with various end groups and PDD-co-TEF; (b) molecular structures of (left) p-BVEs with (─R) (R=─COOH, ─COOCH3, and ─CF3) end groups and (right) molecular structure of PDD-co-TFE. (Figure 3 is reproduced from Refs. [25,27], copyright Japan Society of Applied Physics; and Ref. [30], copyright Wiley-VCH Verlag GmbH.)](/Images/icon/loading.gif)
Fig. 3. (a) Transmittance spectra of p-BVEs with various end groups and PDD-co-TEF; (b) molecular structures of (left) p-BVEs with (─R) (R = ─ COOH , ─ COOCH 3 , and ─ CF 3 ) end groups and (right) molecular structure of PDD-co-TFE. (Figure 3 is reproduced from Refs. [25,27], copyright Japan Society of Applied Physics; and Ref. [30], copyright Wiley-VCH Verlag GmbH.)
![Photographs of the damage to the electrodes after reliability tests using 265 nm LEDs. A similar phenomenon was observed for p-BVE with the ─COOH end group for a 285 nm LED after a reliability test for 804 h [25]. When the damage to the electrodes occurred, a significant increase in the leakage current occurred. (Taken from Ref. [27]; copyright Japan Society of Applied Physics.)](/Images/icon/loading.gif)
Fig. 4. Photographs of the damage to the electrodes after reliability tests using 265 nm LEDs. A similar phenomenon was observed for p-BVE with the ─COOH end group for a 285 nm LED after a reliability test for 804 h [25]. When the damage to the electrodes occurred, a significant increase in the leakage current occurred. (Taken from Ref. [27]; copyright Japan Society of Applied Physics.)
![Bubbles appearing after reliability tests for 32 and 105 h followed by heating up to 230°C for the p-BVE with ─COOH and ─COOCH3 end groups, respectively. The reliability tests were carried out using 265 nm LEDs under an operation current of 20 mA. [Figures 5(a) and 5(c) are taken from Ref. [27]; copyright Japan Society of Applied Physics. Figure 5(b) is taken from Ref. [37]; copyright is shared by the authors and SPIE.]](/Images/icon/loading.gif)
Fig. 5. Bubbles appearing after reliability tests for 32 and 105 h followed by heating up to 230°C for the p-BVE with ─COOH and ─ COOCH 3 end groups, respectively. The reliability tests were carried out using 265 nm LEDs under an operation current of 20 mA. [Figures 5(a) and 5(c) are taken from Ref. [27]; copyright Japan Society of Applied Physics. Figure 5(b) is taken from Ref. [37]; copyright is shared by the authors and SPIE.]
![Damage to the electrodes after reliability tests for 3137 and 832 h at a driving current of 200 mA for 262 nm LEDs wrapped with (a) p-BVE with the ─CF3 end group and (b) PDD-co-TFE. Similar damage to the electrode was observed on a 289 nm LED when using PDD-co-TFE [29]. (Taken from Ref. [29]; copyright Wiley-VCH Verlag GmbH.)](/Images/icon/loading.gif)
Fig. 6. Damage to the electrodes after reliability tests for 3137 and 832 h at a driving current of 200 mA for 262 nm LEDs wrapped with (a) p-BVE with the ─ CF 3 end group and (b) PDD-co-TFE. Similar damage to the electrode was observed on a 289 nm LED when using PDD-co-TFE [29]. (Taken from Ref. [29]; copyright Wiley-VCH Verlag GmbH.)
![TPD-MS spectra for H2O (m/z=18), CO+(N2) (m/z=28), CO2 (m/z=44), and CFO (m/z=47) (a) after the weak irradiation treatment using the 261 nm LED for p-BVE with ─COOH ends and (b) p-BVE with the ─CF3 end group after the strong irradiation treatment, which overlaps those without UV irradiation. Differential FT-IR spectra before and after the 253.7 nm irradiation (c) for p-BVE with the ─COOH end group and (d) for p-BVE with the ─CF3 end group. The irradiation time was 12 h for p-BVE with the ─COOH and ─CF3 end groups. Although the CO (m/z=28) signal of TPD-MS cannot be isolated from the background of N2 (m/z=28), the signal of m/z=28 in (a) has a strong relationship with that of CO2, which enables us to conclude that the peak curve of m/z=28 is mainly due to the CO signal. [Figure 7(a) is reproduced from Ref. [25] with the CFO signal added.]](/Images/icon/loading.gif)
Fig. 7. TPD-MS spectra for H 2 O (m / z = 18 ), CO + ( N 2 ) (m / z = 28 ), CO 2 (m / z = 44 ), and CFO (m / z = 47 ) (a) after the weak irradiation treatment using the 261 nm LED for p-BVE with ─COOH ends and (b) p-BVE with the ─ CF 3 end group after the strong irradiation treatment, which overlaps those without UV irradiation. Differential FT-IR spectra before and after the 253.7 nm irradiation (c) for p-BVE with the ─COOH end group and (d) for p-BVE with the ─ CF 3 end group. The irradiation time was 12 h for p-BVE with the ─COOH and ─ CF 3 end groups. Although the CO (m / z = 28 ) signal of TPD-MS cannot be isolated from the background of N 2 (m / z = 28 ), the signal of m / z = 28 in (a) has a strong relationship with that of CO 2 , which enables us to conclude that the peak curve of m / z = 28 is mainly due to the CO signal. [Figure 7(a) is reproduced from Ref. [25] with the CFO signal added.]
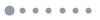
Fig. 8. (a) Decomposition paths for p-BVE with ─COOH end group; (b) alignment of the end group on the electrode surface in the case of ─COOH. The neighboring ─COOH is one of the reasons for the early occurrence of electrode damage in the case of p-BVE with the ─COOH end group.
![(a) TPD-MS spectra of CO2 and CFO for PDD-co-TFE before and after the 261 nm irradiation; (b) differential FT-IR spectrum with a signal of a ketone (>C═O), CFO, and OH after 253.7 nm irradiation for 12 h obtained by subtracting the FT-IR spectrum before the irradiation treatment. The peak at 1890 cm−1 can be assigned to CFO and the increase in the signal intensity at 1860 cm−1 is assigned to a ketone. The peak at 3620 cm−1 is assigned to OH. (Figure 9 is taken from Ref. [29]; copyright Wiley-VCH Verlag GmbH.)](/Images/icon/loading.gif)
Fig. 9. (a) TPD-MS spectra of CO 2 and CFO for PDD-co-TFE before and after the 261 nm irradiation; (b) differential FT-IR spectrum with a signal of a ketone (> C ═ O ), CFO, and OH after 253.7 nm irradiation for 12 h obtained by subtracting the FT-IR spectrum before the irradiation treatment. The peak at 1890 cm − 1 can be assigned to CFO and the increase in the signal intensity at 1860 cm − 1 is assigned to a ketone. The peak at 3620 cm − 1 is assigned to OH. (Figure 9 is taken from Ref. [29]; copyright Wiley-VCH Verlag GmbH.)
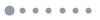
Fig. 10. TPD-MS spectra for (a) N 2 (gray), O 2 (green), and (b) H 2 O (blue) before (hollow symbols) and after (solid triangles indicating increases “▴” and decreases “▾”) in the irradiation treatment using the 261 nm LED. The markedly increased occlusion of N 2 and O 2 is shown by the increased signal intensity without desorption peaks. The H 2 O signal intensity decreased after the irradiation despite the increased occlusion of N 2 and O 2 ; thus, the source of the oxygen to decompose PDD is likely to be H 2 O . The m / z = 28 signal current can be attributed mainly to N 2 .
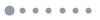
Fig. 11. (Upper) Path for the creation of voids in the case of PDD-co-TFE and (lower) structure after the void creation. The undetectable species due to the high vapor pressure are indicated by “*”.
![Light extraction enhancement ratio (RLEE) plotted against the refractive index for different materials. Red solid circles indicate the cases with a hemisphere sapphire lens [26]. The blue solid circle indicates the case of encapsulation with an unknown resin [11]. The violet solid square indicates the case of fluororesin encapsulation [25,27]. Hollow circles indicate the initial RLEE observed in the experiments by the authors.](/Images/icon/loading.gif)
Fig. 12. Light extraction enhancement ratio (R LEE ) plotted against the refractive index for different materials. Red solid circles indicate the cases with a hemisphere sapphire lens [26]. The blue solid circle indicates the case of encapsulation with an unknown resin [11]. The violet solid square indicates the case of fluororesin encapsulation [25,27]. Hollow circles indicate the initial R LEE observed in the experiments by the authors.
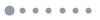
Fig. 13. (a) Photograph of the fabricated lens array on a 50.8 mm × 102.4 mm AlN sheet. (b) Isolated 3535 COS packages. This simple device mount reduces the loss of light resulting from the use of a conventional container-type ceramic package with a quartz window and enhances the light extraction 1.5-fold relative to that of the bare die.
|
Table 1. LEE Enhancement Ratios Obtained Using Encapsulation or a Lensa
|
Table 2. LEE Enhancement Ratios with Reflective Electrodes Are Separately Givena
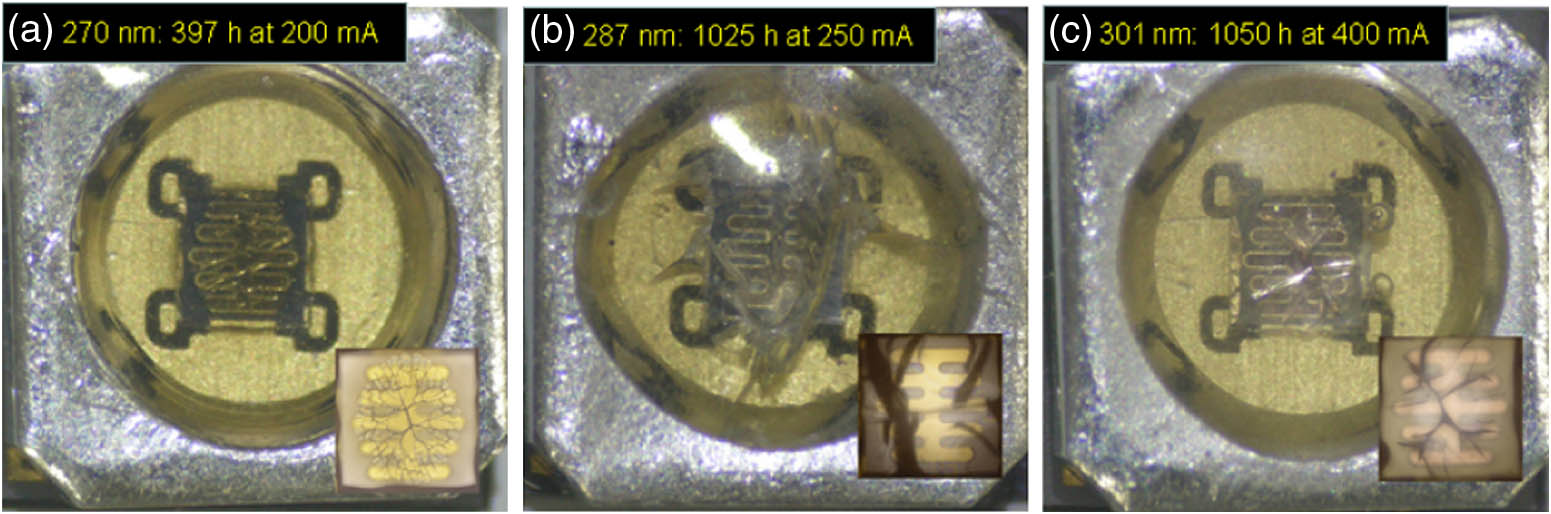
Set citation alerts for the article
Please enter your email address