
- Journal of Inorganic Materials
- Vol. 37, Issue 4, 445 (2022)
Abstract
Nowadays information security plays more and more important roles in the military and economic fields. Among various information protection technologies, the stimuli- responsive luminescence has attracted lots of attention due to low cost and ease of fabrication. Initially, transparent fluorescent materials were directly employed for the confidential information encryption, and the decryption was completed according to the luminescent response of these materials under external stimuli (humidity, temperature and UV light). In addition to relying on those facile signal “switch on-off” determinations, higher-security encryptions were achieved through multimode emission integrated in one single ink[1⇓-3], luminescence lifetime- dependent emission[4⇓-6] and emission intensity-based decoding[7-8]. However, these elaborate emission inks complicate the whole fabrication process and increase the cost of preparation. Besides, the specific equipment is required to decrypt the encoding data, which is certainly inconvenient for the practical applications[9]. Accordingly, the exploration of novel luminescent inks possessing cost advantage and competitive performance are alternatively desirable for the efficient anti-counterfeiting purposes.
Recently, the organic-inorganic hybrid perovskites have emerged as effective photoluminescence materials due to high photoluminescence quantum yields, narrow emission bands and tunable emission spectra[10]. Moreover, the soft ionic structure, low formation energy, together with the low cost facilitate their usage as smart luminescent materials. By space-confining the CsPbBr3 nanocrystals into porous silica matrix, the reversible conversion between luminescent CsPbBr3 and non-luminescent CsPb2Br5 phase can be achieved by using water as the external stimuli. The printed information can be hidden and recovered through moisture treatment and evaporation, respectively[11]. Besides phase transition, Huang, et al.[12-13] reported the in-situ formation and decomposition of luminescent CsPbX3 quantum dots within transparent glasses through femtosecond laser irradiation and thermal treatment, realizing the reversible information writing and erasing. Another attractive technique to realize the information protection and storage was developed by firstly constructing an invisible lead-based metal-organic frameworks and then converting the lead ions to luminescent perovskite nanocrystals with a two-step method. The photoluminescence can be quenched and recovered through solvent impregnation and halide salt conversion. With this strategy, the reversible on/off switching of photoluminescence for information encryption and decryption could be realized under ambient conditions[14].
Herein, we utilize the strong interaction between 2- methylimidazole and lead ions to construct a novel space-confined lead framework (Pb-ZIF). The unique distribution of lead ions within the framework facilitates the in-situ growth of perovskite nanocrystals after reacting with MABr (MA=CH3NH3). The obtained MAPbBr3 NCs@Pb-ZIF exhibits bright luminescence under UV excitation. The recorded information was firstly encrypted by printing the Pb-ZIF ink on parchment paper, which is invisible under ambient and UV light. The subsequent MABr solution spray initializes the in-situ growth of perovskite nanocrystals, making the encrypted information readable under UV light. Using methylammonium bromide and water as the decryption and encryption reagents could switch on/off the luminescence. Different from the direct printing of perovskite nanocrystals, this two-step strategy by separating the confidential information recording and displaying provides the information protection with a higher level.
1 Experimental
1.1 Sample preparation
Pb-ZIF powder was synthesized using a similar modified procedure reported by Sadeghzadeh[15]. Specifically, 6 mmol 2-methylimidazole (Aladdin, 98%) was ultrasonicaly dissolved in 50 mL deionized water (termed as solution A), and then 0.05 mol∙L-1 (20 mL) Pb(NO3)2 (Adamas, 99%) aqueous solution (solution B) was dropwise slowly added into solution A. A clear and transparent solution C was obtained. Finally, an appropriate amount of ethanol (200 mL) was swiftly poured into the above solution C to precipitate Pb-ZIF powders. The obtained powder was rinsed three times with ethanol and then dried at 60 ℃ for further use. For the synthesis of MAPbBr3 NCs@Pb-ZIF powder (similar to the conversion process reported by Zhang[14]), 100 mg Pb-ZIF powder was dispersed in 10 mL n-hexane (Greagent, ≥97%) under constant stirring (termed as suspension D). Then the predissolved MABr (Greatcell Solar) butanol solution (1.5 mL, 20 mg/mL) was rapidly injected, and the white suspension D immediately turned into green emissive suspension E. The as-formed precipitate was then collected and dried (60 ℃) into powder for further characterizations.
1.2 Information coding-decoding procedure
The invisible transparent ink consisting of 1.99 g Pb(NO3)2, 1.48 g 2-methylimidazole and 10 mL N,N- dimethylformamide (DMF, Sigma Aldrich, 99.8%) was loaded into the ink printer (Canon, PIXMA iP2780), and the predesigned various patterns were printed directly on the commercial available paper. Considering the limited amounts of solvent used for each printing procedure, the obtained coding information can be immediately implemented for decoding process. Similar to the conversion process of luminescent MAPbBr3 NCs@Pb-ZIF powders, the spray of MABr butanol solution (20 mg/mL) was employed to realize the in-situ formation of MAPbBr3 NCs at the place where information was recorded.
1.3 Characterization
X-ray diffraction data were collected by using a Bruker D8 Advance powder diffractometer. TEM and corresponding SAED analyses were performed by using JEM-2100F microscope. SEM images and EDS mappings were conducted on JEOL 7800F microscope. Absorption spectra were determined by a Hitachi U-4100 UV-Vis spectrometer. Fluorescence spectrometer (Fluorolog, HORIBA FL-3) was used to record the PL and PL decay curves of the samples. The chemical nature of the powders was investigated by XPS Mg Kα excitation (RBD upgraded PHI-5000C ESCA system, PerkinElmer), the sample of MAPbBr3 NCs@Pb-ZIF was treated with ion etching for 10 s to investigate the internal distribution of the corresponding elements. Thermogravimetric analysis (TGA) was measured on Netzsch STA449F3 instrument (5 ℃/ min, under Argon atmosphere).
2 Results and discussion
A new metal-organic framework (Pb-ZIF) was constructed through the chemical bonding between 2-methylimidazole and lead ions. The MAPbBr3 nanocrystals were in-situ grown within this framework after reacting with MABr (denoted as MAPbBr3 NCs@Pb-ZIF). The detailed synthesis process was presented in the experimental section. The obtained MAPbBr3 NCs@Pb-ZIF powder exhibits bright green emission under UV excitation (Fig. 1). It is noted that the quantum-confinement is directly realized by the metal-organic framework, without the addition of bulky ligands or porous matrix. X-ray diffraction (XRD) patterns of Pb-ZIF and MAPbBr3 NCs@Pb- ZIF powders are shown in Fig. S1. Pb-ZIF shows a sharp and intense peak at ~12.5°, demonstrating the highly crystalline nature of the sample which is further confirmed by well-resolved diffraction spots in the selected area electron diffraction (SAED) pattern (Fig. 2(b)). After reacting with MABr, new peaks at 14.9° and 25.9° are observed, corresponding to the (100) and (111) planes of cubic MAPbBr3 (Pm¯3m space group)[16]. The diffraction peaks of MAPbBr3 nanocrystals are much weaker than those of Pb-ZIF, indicating that the second conversion reaction might mainly occur on the surface of the Pb-ZIF framework, and no bulk perovskite are formed. These results also indicate that MAPbBr3 NCs were in-situ formed within Pb-ZIF framework, and its formation does not dramatically influence the crystal structure of the matrix. Nonetheless, the decreased main peaks intensity of Pb-ZIF along with unshifted diffraction angle implied the redistribution of electron density in the Pb-ZIF structures[17]. Fig. 2(c, d) show the scanning electron microscopy (SEM) images of the Pb-ZIF, which are well-organized flakes with a thicknesses of ~250 nm. After the conversion reaction, the Pb-ZIF framework preserves the original shape but with the generation of small nanoparticles on its surface (Fig. 2(e)). The elemental mapping of the prepared MAPbBr3 NCs@Pb-ZIF (Fig. 2(f)) shows uniform distribution of elements Br and Pb, which further confirms the in-situ formation of the MAPbBr3 perovskite nanocrystals.
Figure S2.Additional optical images of the obtained MAPbBr3 NCs@Pb-ZIF patterns on paper under UV excitation
Figure 2.(a) TEM image and (b) SAED pattern of Pb-ZIF powders SEM images of (c, d) Pb-ZIF and (e) MAPbBr3 NCs@Pb-ZIF powders, and (f) Elemental mappings of MAPbBr3 NCs@Pb-ZIF powder
X-ray photoelectron spectroscopy (XPS) was conducted to investigate the coordination environment of ions in Pb-ZIF and MAPbBr3 NCs@Pb-ZIF powders. Two new peaks assigned to Br3d5/2 and Br3d3/2 are observed at 68.3 and 69.0 eV (Fig. 3(a, c))[18], indicating the insertion of Br ions after the reaction with MABr. Meanwhile, in the high-resolution Pb4f XPS spectrum (Fig. 3(b)), two signals corresponding to Pb4f7/2 and Pb4f5/2 slightly shift to higher binding energy, which suggests that lead ions in MAPbBr3 nanocrystals originate from the Pb-ZIF framework rather than the free Pb ions adsorbe on the surface. No metallic Pb states notorious as carrier trapping centers[19] are detected from high-resolution Pb4f XPS spectrum. It is noted that the loose structure of the Pb- ZIF framework can facilitate the diffusion of MABr and thus promote the conversion of Pb ions encapsulated inside which may account for the evident Br3d signals after ion etching (Fig. 3(d)).
Figure 3.XPS spectra of Pb-ZIF and MAPbBr3 NCs@Pb-ZIF samples (a) Survey XPS spectra; (b) Pb4f XPS spectra (The offset of Pb 4f peaks marked by an arrow); (c) Br3d XPS spectra of Pb-ZIF and MAPbBr3 NCs@Pb-ZIF samples; (d) Br3d XPS spectrum of MAPbBr3 NCs@Pb-ZIF sample after etching
Fig. 4(a) shows the absorption spectra of the Pb-ZIF and MAPbBr3 NCs@Pb-ZIF powders. The absorption of Pb-ZIF is negligible in the whole visible region, while MAPbBr3 NCs@Pb-ZIF possesses a steep absorption edge near 530 nm. The excitonic absorption at 513 nm confirms the strong confinement of perovskite nanocrystals in the MAPbBr3 NCs@Pb-ZIF. Generally the exciton binding energy (Eb) is slightly higher than the thermal energy (RT=26 meV) which results in the domination of free carriers in the MAPbBr3 crystals[20-21]. The notable excitonic feature in this case indicates the strong confinement of the sample. As can be seen from the steady- state photoluminescence (PL) spectrum (Fig. 4(b)), the prepared MAPbBr3 NCs@Pb-ZIF exhibits a sharp and narrow emission at 519 nm with a full width half maximum of only 25 nm under UV excitation. The confinement effect also leads to a blue-shifted PL emission compared with the bulk MAPbBr3 crystals[16]. The PL decay measurement was performed to investigate the recombination kinetics of the MAPbBr3 NCs@Pb-ZIF powder (Fig. 4(c)). With the bi-exponential fitting (Table S1), an average lifetime (τav) of 6.1 ns composed of trap- assisted recombination lifetime (fast decay component, 4 ns) and radiative recombination lifetime (slow decay component, 16.3 ns) was calculated, which is similar to the mesoporous silica confined perovskite phosphor but shorter than the colloidal counterparts[22-23]. Although 2- methylimidazole could act as passivating ligands, the surface or structure defects in the emissive powders still need to be further treated for more efficient radiative recombination, especially within this nanocrystalline regime.
Figure 4.(a) Absorption and (b) steady-state PL spectra of Pb-ZIF and MAPbBr3 NCs@Pb-ZIF samples; (c) PL decay kinetics of MAPbBr3 NCs@Pb-ZIF sample; (d) Schematic confidential information encryption and decryption process based on Pb-ZIF and MAPbBr3 NCs@Pb-ZIF; (e) Optical images of the printed Pb-ZIF and MAPbBr3 NCs@Pb-ZIF patterns under UV excitation
Taking advantage of the invisible feature of the Pb- ZIF intermediate and the strong luminescence of the final perovskite nanocrystals, this two-step strategy shows the potential to be used for information encryption and decryption through simple inkjet printing technology. As shown in Fig. 4(d), the transparent and achromic ink was firstly prepared by dissolving the constituent species, Pb(NO3)2 and 2-methylimidazole, in polar solvent N,N- dimethylformamide (DMF). The mass production of the predesigned patterns could be realized via inkjet printing. To guarantee efficient adhesion and penetration, the commercial paper with fibrous microstructure (Fig. 5(a)) was utilized as the information carrier. Benefitting from the negligible light response of Pb-ZIF (Fig. 4(a) and Fig. S4(a)), the information deposited on the paper is completely invisible under UV and visible light (Fig. 4(e) and Fig. S4(a)), ensuring that the recorded information is faithfully encrypted. After the spray of MABr solution (in n-butanol), the printed Pb-ZIF in the patterned area is locally converted to the luminescent MAPbBr3 nanocrystals. Accordingly, the predesigned information was clearly distinguished. Fig. 4(e) highlights the logo of Shanghai Institute of Ceramics, Chinese Academy of Sciences. Other complex luminescent patterns including QR code, lion dance and the Great Wall are also presented in Fig. 4(e) and Fig. S2, indicating the designability and flexIbility of this strategy.
Figure 5.SEM images of (a) pristine commercial paper, (b) commercial paper after printed with Pb-ZIF ink, (c) commercial paper with MAPbBr3 NCs@Pb-ZIF NCs, (d) representative information encryption and decryption procedure, and (e) PL intensity of the printed MAPbBr3 NCs@Pb-ZIF patterns in the five cycles of encryption and decryption measurement
SEM image (Fig. 5(b)) further reveals that the Pb-ZIF crystals nucleate in the printed areas and are well- distributed on the surface of the substrate. No distinguishable absorption is detected under UV and visible light, providing the encrypted information with a high- level security (Fig. S4(a,b)). After the local conversion to the MAPbBr3 nanocrystals, the printed patterns exhibit strong photoluminescence under UV light (Fig. 4(e)), ensuring that the protected information can be accurately identified. Taking into account the ionic characteristics of the organic-inorganic halide perovskites, the structure could be easily destroyed by polar solvents[24]. As shown in Fig. 5(d), the previously identified QR code no longer emits fluorescence under UV light after the water spray treatment, and thus the information is encrypted. After the water is completely volatilized, the emissive QR code patterns could be regenerated again with the spray of MABr solution. The printed pattern exhibits bright green photoluminescence under UV irradiation (Fig. 5(d)), and the PL intensity maintains 74% of its initial value after five consecutive switching cycles (Fig. 5(e)). In this method, the Pb-ZIF acts as a reservoir of lead source for the circular generation of perovskite nanocrystals, which allows the reversible on/off switching of the photoluminescence and realizes the information encryption and decryption. The prepared Pb-ZIF exhibits good thermal stability (Fig. S5), which is beneficial for the practical applications of this system.
3 Conclusion
In conclusion, we have demonstrated the in-situ conversion of luminescent perovskite nanocrystals from a novel spaced-confined lead-organic framework, which is constructed through the chemical bonding between lead ions and 2-methylimidazole ligand. The unique distribution of lead ions within the framework facilitates the in- situ growth of perovskite nanocrystals after reacting with MABr. This two-step strategy from the invisible intermediate to the photoluminescent nanocrystals is further combined with the inkjet-printing technology to be used for information protection. The invisible Pb-ZIF ink endows the recorded information on parchment paper with a high-security level, and the subsequent MABr solution spray initializes the in-situ growth of perovskite nanocrystals, making the encrypted information readable under UV light. The methylammonium bromide and water can be used as the decryption and encryption reagent to switch on/off the luminescence, thus protecting the recorded confidential information.
Supporting materials
Supporting materials related to this article can be found at
ZHANG Guoqing1,2, QIN Peng1, HUANG Fuqiang1,2,3
(1. State Key Laboratory of High Performance Ceramics and Superfine Microstructure, Shanghai Institute of Ceramics, Chinese Academy of Sciences, Shanghai 201210, China; 2. School of Physical Science and Technology, ShanghaiTech University, Shanghai 200031, China; 3. State Key Laboratory of Rare Earth Materials Chemistry and Applications, College of Chemistry and Molecular Engineering, Peking University, Beijing 100871, China)
Figure S2.Additional optical images of the obtained MAPbBr3 NCs@Pb-ZIF patterns on paper under UV excitation
Figure S2.Additional optical images of the obtained MAPbBr3 NCs@Pb-ZIF patterns on paper under UV excitation
Figure S2.Additional optical images of the obtained MAPbBr3 NCs@Pb-ZIF patterns on paper under UV excitation
Figure S2.Additional optical images of the obtained MAPbBr3 NCs@Pb-ZIF patterns on paper under UV excitation
Figure S2.Additional optical images of the obtained MAPbBr3 NCs@Pb-ZIF patterns on paper under UV excitation
τ1/ns | f1 | τ2/ns | f2 | τavg/ns | |
---|---|---|---|---|---|
Powder | 4.0 | 82.7% | 16.3 | 17.3% | 6.1 |
Table 1.
PL lifetime for MAPbBr3 NCs@Pb-ZIF powders
References
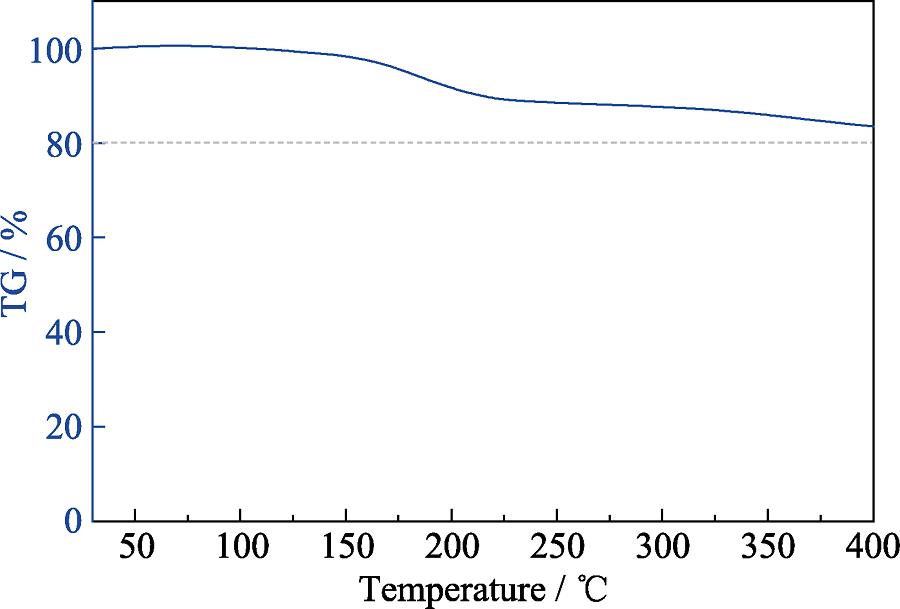
Set citation alerts for the article
Please enter your email address