
- High Power Laser Science and Engineering
- Vol. 4, Issue 3, 03000e30 (2016)
Abstract
1 Introduction
Pulsed x-ray radiography allows the study of fast evolving phenomena like shock compression of matter or plasma outflows. Using short-pulse laser-driven x-ray sources with ps pulse duration helps to reduce smearing of even high velocity phenomena (
Short-pulse (sub-ps to a few ps) high-energy (
X-ray radiography can be achieved in different setups like point-projection geometry, imaging with a pinhole camera or crystal imaging. We used point-projection geometry in our experiment due to its simplicity. In this case, a point like x-ray source projects the sample directly to the detector without use of any imaging system (pinhole camera, spherical crystal or zone plates).
Sign up for High Power Laser Science and Engineering TOC. Get the latest issue of High Power Laser Science and Engineering delivered right to you!Sign up now
Due to the spreading of the electrons in the x-ray source target, the radiation is emitted from an extended zone (reported values range from
A major problem arises in many laser facilities such as LULI2000 or LMJ/PETAL from the geometry of the laser beams (drive beam and backlighter beam) and diagnostic ports. At LULI2000, for example, both laser beams arrive in the equatorial plane of the target chamber at an angle of
Thus, we have chosen a vertical radiography axis (out of the equatorial plane) (see Figure
In this paper we describe the first tests done with this configuration comparing different laser parameters and x-ray sources material with static samples. Then we applied this new scheme to a laboratory astrophysics experiment (POLAR) that has been performed on LULI2000[
2 Setup
The experiment has been performed on the
The x-ray source targets were thin metallic wires pointing in the vertical direction to obtain a good spatial resolution in two dimensions. Target materials were V, Cu, Mo and W with
To characterize the x-ray source, we used several diagnostics. The spatial resolution was measured with a 400 lines per inch,
The detector for the radiography is a
In order to characterize the spectral distribution, we used a crystal spectrometer in von-Hamos geometry covering the K-shell radiation of V and a transmission crystal spectrometer for the Mo K-shell radiation. Thus, for these elements we obtain independent data on the line emission.
3 Results
With the described setup we obtained high-quality radiographs. A typical raw data is shown in Figure
We analyzed the total signal
Signal | Background | Signal | Background | |
---|---|---|---|---|
V | 11.7 | 2.7 | 58 | 4 |
Cu | 3.7 | 0.9 | 11 | 0.6 |
Mo | 9 | 1.5 | 20 | 2.2 |
Table 1. Comparison of x-ray signals from different materials and for two laser wavelengths. The background is measured behind the edge of the gold grid.
We see in all cases a strong increase of the signal using the frequency doubled laser although the laser energy was lower, while the increase of the background signal is less pronounced or even reduced. As the laser intensity was higher in the case of frequency doubling (smaller focus), it is not clear, if this is only due to the laser wavelength and prepulse reduction or if there is also an intensity effect. It is also not clear, which spectral components are responsible for this increase. For V and Mo, where we also measured line radiation, we observed only for Mo an increase in line radiation, which was still less than the spectrally integrated signal on the IP. However, the signal to background ratio was, for frequency doubled laser light, in all cases better.
With changing the laser wavelength, we observed also a strong change in the spectral shape of the emitted x-rays from V samples. While the emission from ionized states (
This is even more surprising, as the laser intensity was higher, which should heat the sample and favorites emission from ionized states[
Using wire targets for radiography along the wire axis, a spatial resolution in the order of the source size, i.e., the diameter of the wire, is expected. We verified this with the grid sample shown in Figure
For the line-out in Figure
We have demonstrated the radiography capabilities in a POLAR experiment, conducted few months later. The Polar experiment consisted to observe the propagation of a high Mach number plasma flow, generated by a strong shock breaking out in the vacuum, and its impact on a solid obstacle (1 mm thick aluminum) located around 2.5 mm away. This target scheme mimics the situation in the so-called POLAR astrophysical objects. Here a main heavy star (white dwarf) accretes matter from a companion star. The matter flow between the stars is collimated due to a high magnetic field (
4 Summary
We have demonstrated successfully reproducible high-quality x-ray radiography in a new configuration of x-ray source and detector. Spatial resolutions better than
References
[6] W. Theobald, K. Akli, R. Clarke, J. A. Delettrez, R. R. Freeman, S. Glenzer, J. Green, G. Gregori, R. Heathcote, N. Izumi, J. A. King, J. A. Koch, J. Kuba, K. Lancaster, A. J. MacKinnon, M. Key, C. Mileham, J. Myatt, D. Neely, P. A. Norreys, H.-S. Park, J. Pasley, P. Patel, S. P. Regan, H. Sawada, R. Shepherd, R. Snavely, R. B. Stephens, C. Stoeckl, M. Storm, B. Zhang, T. C. Sangster. Phys. Plasmas, 13(2006).
[7] H.-S. Park, D. M. Chambers, H.-K. Chung, R. J. Clarke, R. Eagleton, E. Giraldez, T. Goldsack, R. Heathcote, N. Izumi, M. H. Key, J. A. King, J. A. Koch, O. L. Landen, A. Nikroo, P. K. Patel, D. F. Price, B. A. Remington, H. F. Robey, R. A. Snavely, D. A. Steinman, R. B. Stephens, C. Stoeckl, M. Storm, M. Tabak, W. Theobald, R. P. J. Town, J. E. Wickersham, B. B. Zhang. Phys. Plasmas, 13(2006).
[8] R. B. Stephens, R. A. Snavely, Y. Aglitskiy, F. Amiranoff, C. Andersen, D. Batani, S. D. Baton, T. Cowan, R. R. Freeman, T. Hall, S. P. Hatchett, J. M. Hill, M. H. Key, J. A. King, J. A. Koch, M. Koenig, A. J. MacKinnon, K. L. Lancaster, E. Martinolli, P. Norreys, E. Perelli-Cippo, M. Rabec Le Gloahec, C. Rousseaux, J. J. Santos, F. Scianitti. Phys. Rev. E, 69(2004).
[9] K. L. Lancaster, J. S. Green, D. S. Hey, K. U. Akli, J. R. Davies, R. J. Clarke, R. R. Freeman, H. Habara, M. H. Key, R. Kodama, K. Krushelnick, C. D. Murphy, M. Nakatsutsumi, P. Simpson, R. Stephens, C. Stoeckl, T. Yabuuchi, M. Zepf, P. A. Norreys. Phys. Rev. Lett., 98(2007).
[11] H.-S. Park, B. R. Maddox, E. Giraldez, S. P. Hatchett, L. T. Hudson, N. Izumi, M. H. Key, S. Le Pape, A. J. MacKinnon, A. G. MacPhee, P. K. Patel, T. W. Phillips, B. A. Remington, J. F. Seely, R. Tommasini, R. Town, J. Workman, E. Brambrink. Phys. Plasmas, 15(2008).
[14] M. Sonoda, M. Takano, J. Miyahara, H. Kato. Radiology, 148, 833(1983).
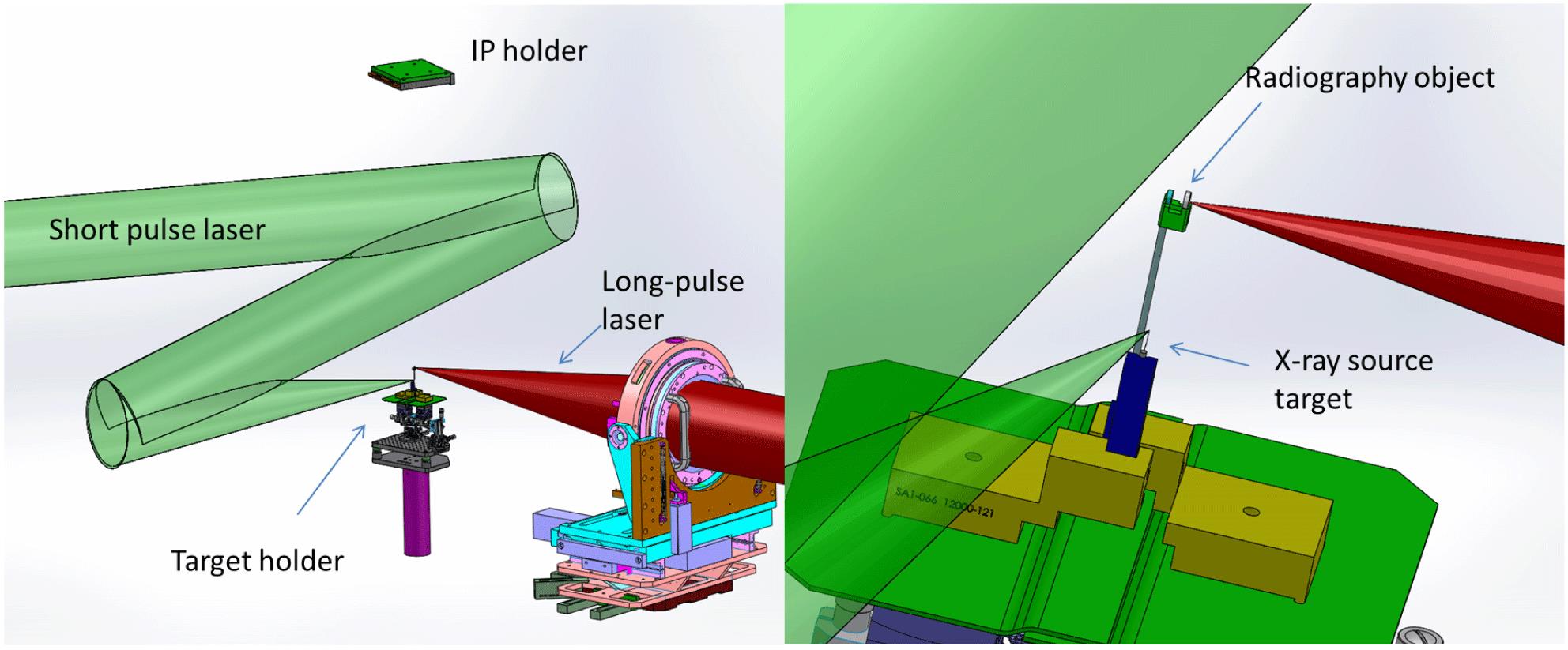
Set citation alerts for the article
Please enter your email address