Author Affiliations
1Max Born Institute for Nonlinear Optics and Short Pulse Spectroscopy, Max-Born-Str. 2a, D-12489 Berlin, Germany2Institute of Chemical Materials, China Academy of Engineering Physics, Mianyang 621900, China3Jiangsu Key Laboratory of Advanced Laser Materials and Devices, Jiangsu Normal University, Xuzhou 221116, China4Department of Physics, Korea Advanced Institute of Science and Technology (KAIST), 34141 Daejeon, South Korea5Física i Cristal·lografia de Materials i Nanomaterials (FiCMA-FiCNA)-EMaS, Departament de Química Física i Inòrganica, Universitat Rovira i Virgili (URV), Campus Sescelades, E-43007 Tarragona, Spain6ITMO University, 49 Kronverkskiy Pr., 197101 St. Petersburg, Russiashow less
Abstract
A mode-locked laser based on a Tm:CNNGG disordered crystal as an active medium and a single-walled carbon nanotube saturable absorber is demonstrated, operating at a central wavelength of 2018 nm. Transform-limited 84 fs pulses are generated with an average output power of 22 mW at a repetition rate of . A maximum output power of 98 mW is obtained at a slightly longer pulse duration of 114 fs.1. INTRODUCTION
Ultrashort pulse laser sources emitting near 2 μm are under rapid development nowadays due to their potential in various applications, e.g., remote sensing, material processing, medical diagnostics, and laser surgery. They present efficient tools for the investigation of the dynamics of physical systems on very short time scales [1], and can be used for synchronous pumping of mid-IR optical parametric oscillators and seeding of near-degenerate optical parametric chirped-pulse amplifiers [2] employed for high-order harmonics generation [3]. However, so far, using Tm- or Ho-doped bulk materials (crystals, ceramics, or glasses), sub-100 fs pulses have been reported only with a crystal (87 fs) and a “mixed” ceramic (63 fs) [4,5]. Therefore, exploring novel solid-state laser media for the generation of ultrashort pulses near 2 μm down to the few-cycle limit is of great importance.
In recent years, disordered host crystals have drawn much attention due to their superior spectral bandwidths and moderate thermal properties. Doped with Tm or Ho ions, they have been proved to be excellent ultrafast laser materials in the 2 μm spectral range [6–12]. In such disordered crystals, different cations can occupy randomly one or more crystallographic sites, resulting in additional inhomogeneous spectral line broadening for the optical transitions of the dopant ion.
Among such disordered hosts, calcium niobium gallium garnets (CNGG) and calcium lithium niobium gallium garnets (CLNGG), with added to partly overcome the nonstoichiometry, have been widely investigated. Active rare-earth ions such as , , and , can be used as dopants to substitute for the ions in dodecahedral sites. The lattice disorder in these type of crystals results from the random distribution of and ions in the octahedral and tetrahedral sites [13]. In the spectral range near 1 μm, pulse durations of 45 and 55 fs were obtained from mode-locked lasers based on Yb-doped CNGG and CLNGG [14,15], respectively, and 534, 660, and 900 fs from Nd-doped CLNGG-CNGG, CNGG, and CLNGG garnets, respectively [16–18]. In the 2 μm spectral range, such disordered crystals doped with have shown broad and smooth continuous-wave (CW) laser tuning, which is very promising for sub-100-fs pulse generation [19]. However, only Tm:CLNGG has been investigated for mode locking: pulse durations of 354 and 479 fs were obtained applying graphene or a semiconductor saturable absorber mirror as saturable absorbers [10,11]. Considering that CNGG and CLNGG have similar spectral properties to other dopants, we decided to study the mode-locked laser performance of Tm:CNGG. We additionally introduced into the crystal (hereafter Tm:CNNGG) to decrease the temperature of crystal growth and promote incorporation [13,20].
Sign up for Photonics Research TOC. Get the latest issue of Photonics Research delivered right to you!Sign up now
In this work, we present the CW and mode-locked laser performance of Tm:CNNGG. Applying a single-walled carbon nanotube saturable absorber (SWCNT-SA) and chirped mirrors for dispersion compensation, the mode-locked Tm:CNNGG laser generated pulses as short as 84 fs at 2018 nm.
2. EXPERIMENTAL DETAILS AND DISCUSSION
The Tm:CNNGG disordered crystal used in the experiment was grown by the conventional Czochralski method with a doping of 3.2 at. % and doping of 5.5 at. %, corresponding to a concentration of and (measured in the crystal), respectively. For the growth process, was selected as the composition to maintain charge neutrality. The maximum absorption cross-section was at 785.8 nm ( transition) and the full width at half maximum (FWHM) of this band amounted to 29 nm. The emission due to the transition extended from 1600 to 2050 nm. The highest emission cross-section, , was at 1866.8 nm [20].
Figure 1 shows the calculated gain cross-section . It can be seen that the gain maximum of Tm:CNNGG is located at wavelengths below 2000 nm for , where strong water vapor absorption in air will prevent stable femtosecond mode locking. Only for low population inversion levels, i.e., , is lasing in Tm:CNNGG expected around 2000 nm. Such wavelengths are long enough to intrinsically avoid the strong water vapor absorption. This, together with the broad and smooth gain characteristics of the spectrum, is a prerequisite for femtosecond pulse generation.
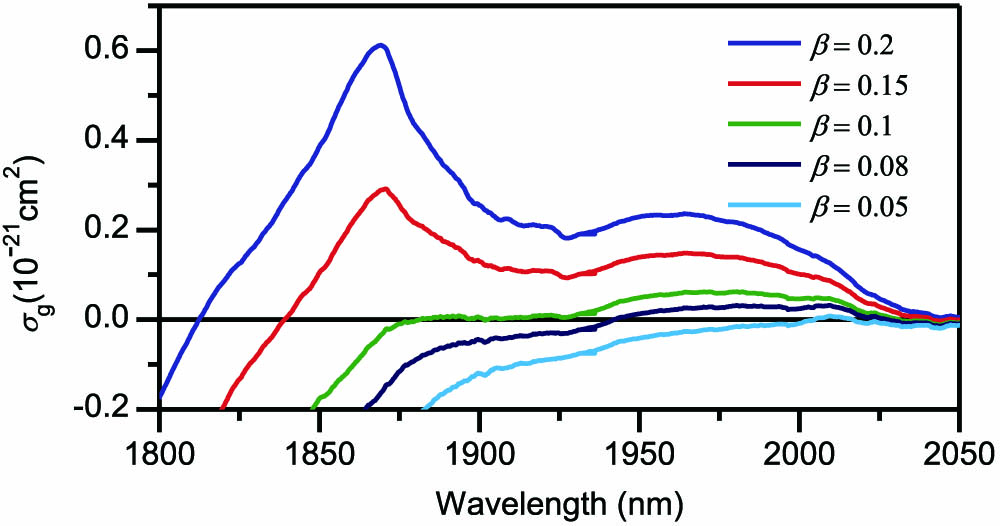
Figure 1.Gain cross-section for the transition of in CNNGG for different inversion levels .
In order to investigate the mode-locked laser performance of Tm:CNNGG, a standard X-shaped cavity as shown in Fig. 2 was employed. The sample used was 3.5 mm long, with a cross-section of . Both end faces of the crystal, which were perpendicular to the (111) crystallographic direction, were optically polished. The sample was placed at Brewster’s angle between two folding concave mirrors and (radius of curvature, ). To remove the generated heat during laser operation, the crystal was wrapped with indium foil and tightly mounted in a water-cooled copper block. The cooling water was maintained at a temperature of 14.5°C. The pump source applied was a narrow-band CW Ti:sapphire laser with a maximum output power of 3.6 W at 785.8 nm. The output beam of the Ti:sapphire laser was focused into the Tm:CNNGG crystal with a spot radius of using a focusing lens with a focal length of 70 mm. Four chirped mirrors (Layertec GmbH, Germany), CM1–CM4, were employed for intracavity dispersion compensation, each of them providing a group delay dispersion per bounce. The intracavity GDD was varied by the number of bounces on the two plane chirped mirrors ().
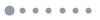
Figure 2.Scheme of the mode-locked Tm:CNNGG laser (L, lens; , dichroic folding mirrors; , plane mirror; CM1–CM4, chirped mirrors; OC, output coupler).
Initially the CW laser performance was investigated with four different output couplers (OCs) having a transmission T of 0.2%, 0.5%, 1.5%, and 3.0%, employing mirror and without the chirped mirrors (see Fig. 2). The total cavity length was . Figure 3(a) shows the output power versus the absorbed pump power. Maximum output powers of 124, 204, 237, and 193 mW were achieved with 0.2%, 0.5%, 1.5%, and 3.0% OCs, respectively. The maximum slope efficiency reached 43.5%. It should be noted that the output power increases slower than the linear dependence at the maximum applied pump levels, indicating an onset of thermal effects; thus, the full available pump power was not employed in this experiment.
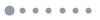
Figure 3.(a) Output power versus absorbed pump power with different OCs in CW operation, and (b) wavelength tuning using a Lyot filter and OC with transmission . In (a), denotes the slope efficiency with respect to the absorbed pump power.
Wavelength tuning of the Tm:CNNGG laser was studied by inserting a Lyot filter into the cavity, close to the output coupler (see Fig. 2). The Lyot filter was a 3.2 mm thick quartz plate with the optical axis at 60° to the surface. Figure 3(b) shows the tuning performance obtained with the OC: a wavelength tuning range of 207 nm (from 1879 to 2086 nm) was achieved, indicating the great potential of the Tm:CNNGG disordered crystal for the generation of ultrashort laser pulses.
To study the mode-locked laser performance, a transmission-type SWCNT-SA was inserted under Brewster’s angle in the vicinity of the second waist formed by and . This SA was characterized by a modulation depth of , a saturation fluence of , and an interband carrier relaxation time of around 2.0 μm [21]. Based on the ABCD propagation matrix method, the waist radius of the laser mode on the SWCNT-SA was calculated to be about 100 and 65 μm in the tangential and sagittal planes, respectively. With two bounces on each of the two plane chirped mirrors and (cf. Fig. 2), the total number of bounces on the chirped mirrors amounted to 11 per round trip. Taking into account the contribution of the 3.5 mm thick crystal under Brewster’s angle and the 1 mm thick SA fused silica substrate, the total round trip GDD was calculated and details are shown in Fig. 4. Around 2000 nm, the total round trip GDD is about .
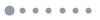
Figure 4.Total round trip GDD introduced by chirped mirrors, Tm:CNNGG, and fused silica substrate of the SA.
At first, an OC with was used. The pump power was set at 3.11 W, corresponding to an absorbed pump power of 1.15 W. After carefully optimizing the separations of and and adjusting the position of the SWCNT-SA and Tm:CNNGG crystals, stable and self-starting mode-locked operation was achieved. Pulses as short as 114 fs (FWHM intensity under shape assumption) were obtained. Figure 5(a) shows the corresponding autocorrelation trace measured with a resolution of 8.4 fs. The corresponding optical spectrum shown in Fig. 5(b) was recorded with an optical spectrum analyzer (resolution: 0.3 nm); it has an FWHM of 37.3 nm centered at 1994 nm. This leads to a time-bandwidth product (TBP) of 0.321, corresponding to nearly Fourier-limited pulses. The measured average output power of the mode-locked laser was 98 mW. From the calculated intracavity power, an average fluence of on the SA was estimated.
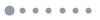
Figure 5.Recorded autocorrelation traces (raw data and fits) and optical spectra with (a), (b) , (c), (d) , and (e), (f) (e–f).
With the aim of achieving shorter pulses, OCs with lower transmission, and 0.2%, were further applied. Pulses as short as 92 and 84 fs, respectively, were obtained with these OCs [see Figs. 5(c) and 5(e)]. The spectral FWHM bandwidths of 49.3 nm centered at 2013 nm [Fig. 5(d)] and 52.8 nm centered at 2018 nm [Fig. 5(f)] resulted in TBPs of 0.335 and 0.327, respectively. These TBPs also indicate that the mode-locked pulses were nearly bandwidth limited at shorter durations. The corresponding average output powers were 40 and 22 mW, and the average fluence on the SA was and . No damage to the SWCNT-SA was observed at this fluence level. The applied pump level was the same for all OCs (corresponding to of absorbed pump power) because the pulses tended to shorten with the pump level even in the region of slight thermal roll-off in Fig. 3(a), as long as the pump beam mode quality was excellent.
Compared to the previous results obtained with a Tm-doped CLNGG [11], much shorter pulses were achieved in the present experiment. The central wavelength in the mode-locked regime is similar to Ref. [11], which is an indication of similar overall cavity losses. Using a Ti:sapphire pump laser with high brightness and beam quality normally creates better conditions for Kerr lensing; however, pure Kerr-lens mode locking was not achievable in our laser without the SA, which obviously played a crucial role for the self-starting and self-stabilization of the mode-locked regime.
The stability of the mode-locked regime was also assessed by measurements with a radio-frequency (RF) spectrum analyzer. The RF spectrum shows an extinction ratio above the noise level of for the fundamental beat note, measured with a resolution bandwidth of 300 Hz [see Fig. 6(a)]. The uniform harmonic beat notes recorded in Fig. 6(b) indicate stable mode locking without any switching or other instabilities. The pulse repetition rate of corresponds to a laser cavity length of . The results were similar for the different OCs.
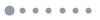
Figure 6.Radio frequency spectra of the mode-locked Tm:CNNGG laser with the 1.5% OC: (a) fundamental beat note and (b) 1.0 GHz wide-span (RBW: resolution bandwidth).
As the output coupler transmission was decreased to 0.5% and 0.2%, stimulated Raman scattering sidebands were observed in the laser spectrum. They can be seen in the spectra shown in Figs. 5(d) and 5(f). The first Stokes component was clearly observed at 2134.5 nm (with ) and 2144.1 nm (with ), at similar separation from the corresponding fundamental central laser wavelengths. Simultaneously, the first anti-Stokes component was also observed (see the same figures), although its intensity was much lower. These sidebands can be well explained by the Raman spectrum of Tm:CNNGG [20]. The first Stokes and anti-Stokes components are generated in the CNNGG crystal by impulsive stimulated Raman scattering with the mode centered at .
3. CONCLUSION
In conclusion, CW, tunable, and passively mode-locked laser operation were studied for the first time to our knowledge with a disordered Tm:CNNGG garnet crystal. A maximum slope efficiency of 43.5% and wavelength tuning range of 207 nm were achieved in the CW regime. Introducing an SWCNT-SA, the Tm:CNNGG laser generated nearly Fourier-limited pulses as short as 84 fs with an output power of 22 mW at a repetition rate of . The stable and self-starting mode-locked laser performance is evidenced by the remarkably high extinction ratio of 78 dB above the carrier of the fundamental beat note in the RF spectrum. To the best of our knowledge, these results represent the first sub-100 fs mode-locked laser operation achieved with a garnet crystal around 2 μm, as well as the shortest pulses ever achieved with Tm-doped crystalline materials.
References
[1] U. Keller. Ultrafast solid-state laser oscillators: a success story for the last 20 years with no end in sight. Appl. Phys. B, 100, 15-28(2010).
[2] V. Petrov. Frequency down-conversion of solid-state laser sources to the mid-infrared spectral range using non-oxide nonlinear crystals. Prog. Quantum Electron., 42, 1-106(2015).
[3] C. Lai, K. Hong, J. P. Siqueira, P. Krogen, C. Chang, G. J. Stein, H. Liang, P. D. Keathley, G. Laurent, J. Moses, L. E. Zapata, F. X. Kärtner. Multi-mJ mid-infrared kHz OPCPA and Yb-doped pump lasers for tabletop coherent soft x-ray generation. J. Opt., 17, 094009(2015).
[4] Y. Wang, W. Chen, M. Mero, L. Zhang, H. Lin, Z. Lin, G. Zhang, F. Rotermund, Y. J. Cho, P. Loiko, X. Mateos, U. Griebner, V. Petrov. Sub-100 fs Tm:MgWO4 laser at 2017 nm mode locked by a graphene saturable absorber. Opt. Lett., 42, 3076-3079(2017).
[5] Y. Wang, W. Jing, P. Loiko, Y. Zhao, H. Huang, S. Suomalainen, A. Härkönen, M. Guina, X. Mateos, U. Griebner, V. Petrov. Sub-10 optical-cycle passively mode-locked Tm:(Lu2/3Sc1/3)2O3 ceramic laser at 2 μm. Opt. Express, 26, 10299-10304(2018).
[6] A. A. Lagatsky, X. Han, M. D. Serrano, C. Cascales, C. Zaldo, S. Calvez, M. D. Dawson, J. A. Gupta, C. T. A. Brown, W. Sibbett. Femtosecond (191 fs) NaY(WO4)2 Tm, Ho-codoped laser at 2060 nm. Opt. Lett., 35, 3027-3029(2010).
[7] J. M. Cano-Torres, M. Rico, X. Han, M. D. Serrano, C. Cascales, C. Zaldo, V. Petrov, U. Griebner, X. Mateos, P. Koopmann, C. Kränkel. Comparative study of crystallographic, spectroscopic, and laser properties of Tm3+ in NaT(WO4)2 (T=La, Gd, Y, and Lu) disordered single crystals. Phys. Rev. B, 84, 174207(2011).
[8] Y. Wang, G. Xie, X. Xu, J. Di, Z. Qin, S. Suomalainen, M. Guina, A. Härkönen, A. Agnesi, U. Griebner, X. Mateos, P. Loiko, V. Petrov. SESAM mode-locked Tm:CALGO laser at 2 μm. Opt. Mater. Express, 6, 131-136(2016).
[9] L. Kong, Z. Qin, G. Xie, X. Xu, J. Xu, P. Yuan, L. Qian. Dual-wavelength synchronous operation of a mode-locked 2-μm Tm:CaYAlO4 laser. Opt. Lett., 40, 356-358(2015).
[10] J. Ma, G. Xie, P. Lv, W. Gao, P. Yuan, L. Qian, U. Griebner, V. Petrov, H. Yu, H. Zhang, J. Wang. Wavelength-versatile graphene-gold film saturable absorber mirror for ultra-broadband mode-locking of bulk lasers. Sci. Rep., 4, 5016(2014).
[11] J. Ma, G. Xie, W. Gao, P. Yuan, L. Qian, H. Yu, H. Zhang, J. Wang. Diode-pumped mode-locked femtosecond Tm:CLNGG disordered crystal laser. Opt. Lett., 37, 1376-1378(2012).
[12] J. Ma, G. Xie, P. Lv, W. Gao, P. Yuan, L. Qian, H. Yu, H. Zhang, J. Wang, D. Tang. Graphene mode-locked femtosecond laser at 2 μm wavelength. Opt. Lett., 37, 2085-2087(2012).
[13] E. Castellano-Hernández, M. D. Serrano, R. J. Jiménez Riobóo, C. Cascales, C. Zaldo, A. Jezowski, P. A. Loiko. Na modification of lanthanide doped Ca3Nb1.5Ga3.5O12-type laser garnets: Czochralski crystal growth and characterization. Cryst. Growth Des., 16, 1480-1491(2016).
[14] J. Ma, Z. Pan, J. Wang, H. Yuan, H. Cai, G. Xie, L. Qian, D. Shen, D. Tang. Generation of sub-50 fs soliton pulses from a mode-locked Yb, Na:CNGG disordered crystal laser. Opt. Express, 25, 14968-14973(2017).
[15] Y. Zhang, V. Petrov, U. Griebner, X. Zhang, H. Yu, H. Zhang, J. Liu. Diode-pumped SESAM mode-locked Yb:CLNGG laser. Opt. Laser Technol., 69, 144-147(2015).
[16] G. Xie, L. Qian, P. Yuan, D. Tang, W. Tan, H. Yu, H. Zhang, J. Wang. Generation of 534 fs pulses from a passively mode-locked Nd:CLNGG-CNGG disordered crystal hybrid laser. Laser Phys. Lett., 7, 483-486(2010).
[17] G. Xie, D. Tang, H. Luo, H. Zhang, H. Yu, J. Wang, X. Tao, M. Jiang, L. Qian. Dual-wavelength synchronously mode-locked Nd:CNGG laser. Opt. Lett., 33, 1872-1874(2008).
[18] G. Xie, D. Tang, W. Tan, H. Luo, H. Zhang, H. Yu, J. Wang. Subpicosecond pulse generation from a Nd:CLNGG disordered crystal laser. Opt. Lett., 34, 103-105(2009).
[19] W. Gao, G. Xie, J. Ma, M. Liu, P. Yuan, L. Qian, H. Yu, H. Zhang, J. Wang, J. Zhang. Spectroscopic characteristics and efficient laser operation of Tm:CLNGG disordered crystal. Laser Phys. Lett., 10, 055809(2013).
[20] Z. Pan, J. Serres, E. Kifle, P. Loiko, H. Yuan, X. Dai, H. Cai, M. Aguiló, F. Díaz, Y. Wang, Y. Zhao, U. Griebner, V. Petrov, X. Mateos. Crystal growth and comparative study of spectroscopic and laser properties of Tm3+, Na+(Li+)-codoped Ca3Nb1.5Ga3.5O12-type disordered garnet crystals. Opt. Express(2018).
[21] W. Cho, A. Schmidt, J. Yim, S. Choi, S. Lee, F. Rotermund, U. Griebner, G. Steinmeyer, V. Petrov, X. Mateos, M. Pujol, J. Carvajal, M. Aguilo, F. Diaz. Passive mode-locking of a Tm-doped bulk laser near 2 μm using a carbon nanotube saturable absorber. Opt. Express, 17, 11007-11012(2009).