Abstract
Professor Marlan Scully discusses his career in quantum optics with the Editor-in-Chief of Photonics Research, Prof. Lan Yang.The first interview for a Photonics Research webinar series took place on 26 May 2021. Editor-in-Chief, Prof. Lan Yang interviewed Prof. Marlan Scully, Texas A&M University, United States. He is a world leader in the field of quantum optics and has made numerous groundbreaking discoveries that include the first quantum theory of lasers with Willis Lamb, the first demonstration of lasing without inversion, and the first demonstration of ultra-slow light in hot gases, just to name a few. He also published two classic textbooks, Quantum Optics coauthored with Suhail Zubairy and Laser Physics coauthored with Murray Sargent and Willis Lamb. They are invaluable references for anyone wanting to know more about quantum optics and laser science. He has been elected to the National Academy of Sciences, the National Academy of Inventors, the American Academy of Arts and Sciences, Academia Europaea, the Russian Academy of Sciences, and the Max Planck Society. He was recently elected as a Foreign Member of Chinese Academy of Sciences. Excerpts from the interview are provided below. The full transcript is available in the video section at www.researching.cn/pr/highlights.
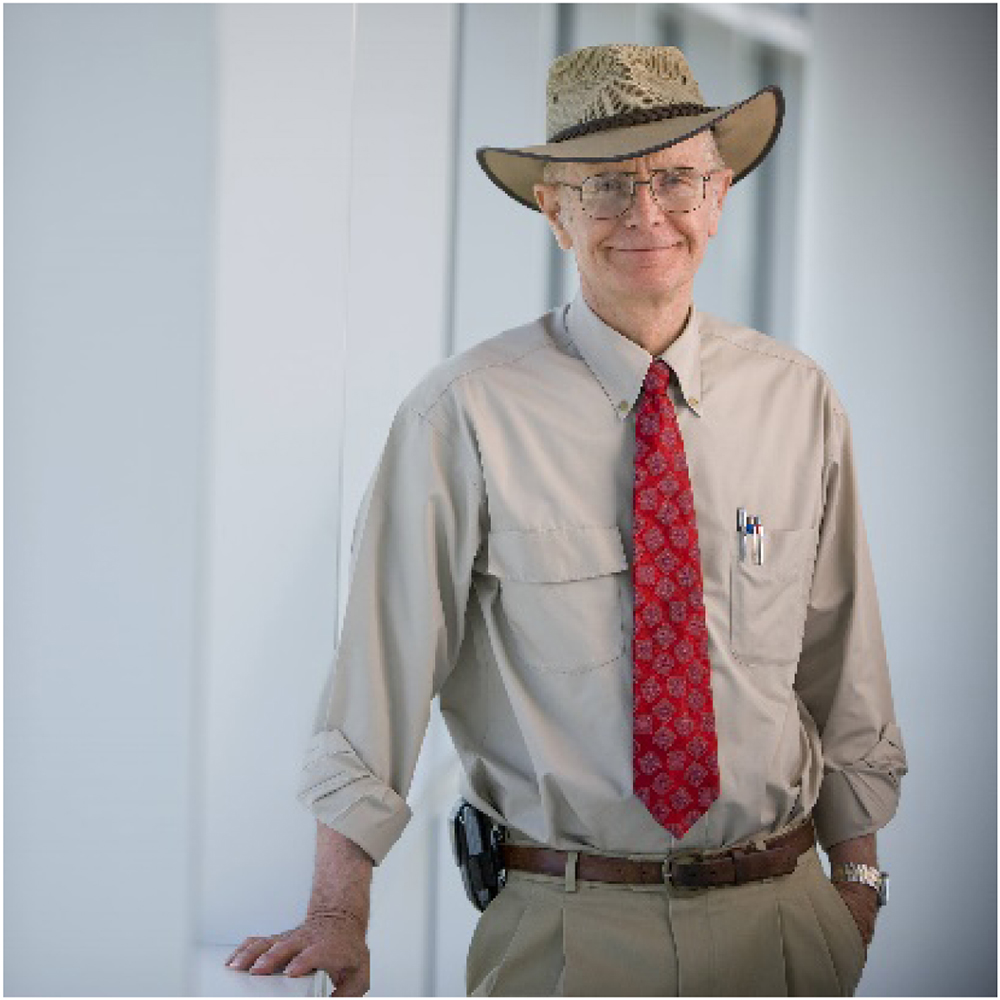
Figure 1.Prof. Marlan Scully, Texas A&M University, United States.
Lan Yang: You have been in the area of quantum optics for many decades. How did you get involved in this field?
Marlan Scully: I was working in the field of low temperature physics when the laser really started coming into its own. I was at Yale where Willis Lamb, of the Lamb shift, opened up quantum electrodynamics as a real field. I was doing the grading for his course in quantum mechanics. I had been fortunate to have quantum mechanics as an undergraduate at the University of Wyoming. A small school, but a jewel of a physics department and a wonderful Professor named Bob Bessey. Eventually, at Yale I realized that low temperature physics is hard. Lamb suggested that I work with him to understand the fundamentals of lasers, such as, how do we map the laser density matrix from below threshold to above threshold. Lamb suggested this problem to me and thought there would be enough for a thesis. Lucky me. I had Willis Lamb and such an interesting problem in its early days. That’s how I got into quantum optics, good luck and great people to work with.
Sign up for Photonics Research TOC. Get the latest issue of Photonics Research delivered right to you!Sign up now
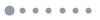
Figure 2.Left to right: Ali Javan, Paul Mandel, Marlan Scully, Willis Lamb, and Olga Kocharovskaya (provided by Marlan Scully).
Lan Yang: What is the most important impact of quantum optics to our daily life?
Marlan Scully: Certainly, the laser has been an unparalleled tool of great boon to us. And the transistor and the computer are other examples, but doing laser spectroscopy and doing optics is something that has opened up after the laser. When I was a student in the 1960s, optics was not a particularly exciting area. There was lots of interesting stuff, like Fabry–Pérot cavities and looking at spectra, but it wasn’t a hot area.
Along comes the laser. Wow. Now, we can do all kinds of spectroscopy. Think about Raman spectroscopy. Here we can look at the light, which is generated by an incident laser, and it is then bouncing off of a molecule, which is oscillating, so that the reflected light will have generally lower frequency. Downshifted. Stokes radiation, it’s called. Oh, by the way, the guy who suggested this was a theorist. The early days of Smekal’s work, before quantum mechanics, 1924. It was a great theoretical idea. But then, along came Raman and he did the experiments and understood the physics and used sunlight. But only one photon in is converted into a Raman-shifted photon. Wow, not much light, but with the laser, now you get a good signal. People like Boris Stoicheff in Canada were heroes in using the laser in this Raman spectroscopy. And, oh, by the way, when Stoicheff went to India, his spectral signature was there on Raman’s wall.
So that’s certainly an example of the fact that the laser has opened up this whole field of optics. Nonlinear optics, quantum optics, laser spectroscopy. What a great field this is today.
Lan Yang: Talking about spectroscopy, what you just said links to my next question. In addition to quantum optics, you made impressive contributions to sensing and spectroscopy technologies. For example, you have papers about the detection of anthrax spores by using femtosecond adaptive spectroscopic technologies and coherence Raman microscopy. What was the motivation to investigate this? Is there any story behind that?
Marlan Scully: The question of how we address problems in our field and beyond our field is a very important question, and I always encourage students to approach applied physics and fundamental physics equally, so that you’re interested in questions, for example, of general relativity and looking at how you can probe black holes or gravitational radiation detected by a laser interferometer on the one hand. But looking at other important problems that are on the horizon, back in the early days of the anthrax murders in Princeton of 2001, it was very clear that we needed a technique to detect anthrax much faster than the laboratory chemistry experiments or techniques that were used to decide whether some white powder of it coming out with a mail was anthrax.
So, I began looking at this problem and just decided that we had a chance to use Raman spectroscopy. But Raman spectroscopy is still weak, as I said before, one photon in ; that’s not enough signal. How about using coherent Raman? What do you mean “coherent”? I’ve got an ensemble of molecules and they’re in the sample. Now I’ve got molecules, a little sample that came from the mail. Now, if I can somehow orchestrate these molecules so they’re oscillating coherently, then I can increase the signal by a factor of N (the number of molecules). So, with ordinary Raman, the intensity comes in proportion to the number of items per milliliter. But with coherent anti-Stokes Raman spectroscopy (CARS), we can get the signal going like . That’s a nice advantage. Unfortunately, you also begin to pick up the background and that clouds the signal, so that you’re not able to really do what you want, with ordinary coherent Raman. Extraordinary coherent Raman, if you will. Extraordinary, because it goes like . Yep, that’s the good news. The bad news is that the noise also goes like (Nbg is the number of background molecules) and you can’t tell them apart. So, I began looking at ways in which we could improve CARS, and, in particular, came up with this improved technique which we called femtosecond adaptive spectroscopic techniques for CARS, i.e., FAST CARS.
It turned out that had some promise, so I went to DARPA and told them what we were thinking, and they said, “Oh, that won’t work. But it’s a very important problem. So, here’s a million dollars, go find out why it won’t work.” I went back to Texas and got together with some of our guys, and we decided that we can show them why it very likely would work. We went back a little bit later and they said, “Yeah, that’s great. Here’s $10 million. Now go do the experiments.” Well, we needed femtosecond lasers, but we didn’t have them. Indeed, and in those times, in the early part of the century, the femtosecond lasers were available at a few labs like Michigan and like Princeton. So, I called my friends at Princeton, where I have a lot of colleagues. I said, “Here’s what we’re doing. What do you think?” And they said, “Well, we’ve got so many people who want to use these lasers, that only our faculty can use them, but if you’d be willing to come as a visiting professor, we’ll talk it over.”
So, I went for a “job interview” and I presented a technique. A guy in the back of the room jumped up and he said, “This is crazy. You’re wrong. It’s well known that CARS doesn’t work because you have to amplify the noise blah blah blah.” Well, I didn’t want to argue with the guy. I wanted to use his laser, right? I said, “Well, I always want my strongest criticism from my friends in private, not my enemies in public. So, thank you for your question.” He says, “Well, I count 200 people here in the room. This is not private and we’re not your friends.” (Laugh) I go on with my case. I just have to say, “You’re wrong and here’s why.” I explained my position, and he and I argued for a week, and I finally converted him into a believer. We worked on the problem for a couple of years at Princeton and at A&M. We were learning to use and make these lasers. And my friends here at A&M, Alexi Sokolov and some of the bright students actually got it going.
The key point is that anthrax is 17% by weight of something called calcium dipicolinate. Picture benzene, knockout one of the carbon atoms, put in a nitrogen called pyridine, and now attach two carboxylic acid molecules, COOH. You get the picture that you’ve got something simple: 16, 17 atoms in this molecule. The number of spectral lines is not overwhelming.
We went through the ropes of learning how to do this FAST CARS and before we were done we could take a sample of anthrax white powder to do ordinary Raman measurements, which people at Princeton had learned back in the 1970s. Takes five minutes, maybe, maybe a little less, maybe a minute, but takes a long time. And here, you’d like to be able to probe an envelope as it sweeps through the mail at a millisecond or 10 milliseconds or some such time. Well, we finally got this FAST CARS technique working so that we could spot anthrax in a nanosecond. Maybe a microsecond, even, but very fast. That kind of advance in the utility of this technique led us to look at other ways to apply CARS and FAST CARS to spectroscopy such that, when the COVID problem came along, we were there right away. And had the good fortune to be working with our German colleagues. A guy named Volker Deckert was here with us and had many interesting ideas about how we could use these techniques to advantage. We did, in fact, we learned to make the FAST go with enhanced resolution, so he suggested, “Let’s call it FASTER CARS.” That’s indeed what we did.
Using these techniques now to spot a single COVID 19 virus and to scan out using tip-enhanced Raman spectroscopy, another bit of jargon. But take a very tiny tip like you see in the atomic force microscope. You run this tiny tip across the surface and do Raman measurements of the amino acids on the surface and we get great resolution.
To make a long story long that’s the anthrax activity going on here in our lab.
Lan Yang: Thanks for sharing your amazing story. What were your reasons to start the Winter Colloquium on the Physics of Quantum Electronics, which is also known as PQE? I have attended this conference a couple of times and was truly impressed by the quality of the talks.
Marlan Scully: Well, I began working with people at Los Alamos and at Kirtland Research Laser Lab there in Albuquerque (New Mexico). They were all great skiers. They thought it would be really great if we could have a meeting at someplace interesting like this new Snowbird, Utah, slope that was opening up. We could go there and go to meetings in the morning, ski in the afternoon, and then come back and go to meetings in the evening. So, we did that. Well, it was a big hit. Not only was it fun, it was also an excellent way to do science, because you’re tired after, say, lectures from 8:00 AM till 11:30 AM. So, if you can go out and ski and you’re sitting in a ski lift next to, say, Julian Schwinger (that was the highlight of my PQE activity), you can talk to them in a relaxed mode and you can enjoy the opportunity to argue in a very friendly and excellent environment. We continued doing this and 50 years later we’re still doing it too. PQE has been quite successful, and we’ve had people who’ve come to the conference and gone on to win the Nobel Prize, e.g., Glauber and Ketterle, and many guys who are now leaders in our field. It’s a kind of community, a kind of a family of people who join us each year. Now it’s 200 or 300 (attendees) and we get to know each other in ways that we couldn’t or wouldn’t if we were simply meeting at the New York Physical Society meeting.
Lan Yang: Would you like to say something about your current research interests?
Marlan Scully: I can answer that by saying what I was doing before COVID hit us. I was working on the problem which was brought to me by David Lee, Nobel Prize for showing that helium-3 can be superfluid. I was fortunate to hire him here at Texas A&M. David came in one day and said, “How come the Hawking entropy of a black hole is proportional to the area of the black hole and not the volume?” I thought that was a great question. I didn’t know the answer and wanted to work it out. I looked in the textbooks and it wasn’t really there the way I wanted to see it, so I worked out an alternative point of view, which used Unruh radiation. Bill Unruh was one of the people who gave us deep insights into black hole radiation after Hawking. I used Bill’s techniques and came up with a new way to understand black hole radiation, by looking at light that an atom emits as it falls into a black hole. Well, I’m fascinated.
That’s one example of what we’re interested in and what I would like to do much more of if I can find some spare time to work on this. And to look at the way in which, let me say this slowly, the way in which quantum optics and squeezed light have a very deep connection to this kind of black hole, Hawking Unruh radiation. Squeezed light. Entanglement. Black hole radiation. Hawking radiation. Gee, it’s entangled! Isn’t that neat. How can that be? I’d like to advertise this book, Quantum Optics, with Suhail Zubairy, my wonderful colleague down the hall. Here is an example of ways in which quantum optics, and this is theoretical quantum physics, is able to interface with, contribute to in some way, be enriched by in a deeper way, a field as remote as general relativity and black hole physics. So that’s one thing that I’m very interested in. I hope to get back to that, but I still feel like we haven’t finished our job with the COVID-19 problem.
Lan Yang: What would be your advice to young researchers who want to pursue research in quantum optics? What background knowledge should be acquired before diving into it?
Marlan Scully: First, find a problem that you’re really interested in. Don’t go into some area because you think it’s going to make you wealthy. Find something that really interests you. In quantum optics we have a huge array of problems to choose from. We have problems which are very fundamental. Entanglement. How can we use squeezed light to advantage, to make a better microscope? These are problems which, on the one hand, are very fundamental. On the other hand, they have applications. Whatever your main interest is, think about ways in which you can apply these techniques to advantage and make a good living. In my case, I was interested in lasers. I found early on that the laser gyroscope was of interest in industry and I spent decades consulting on the laser gyroscope. It was not so interesting to me that I would have done that, as my first choice of problems, but it was very interesting once we got into it. Get something going which you really like and you’d like to understand better. For example, how is it that we can use entangled radiation to advantage in making a spectroscopic measurement, making a Raman measurement? And then, how can I apply these ideas in detecting single viruses or single cancer elements.
Lan Yang: Could you tell us about the worldwide competition and collaboration in the early days of understanding of the physics of lasers, especially the quantum statistical nature of lasers and also how it is different from spontaneous emission?
Marlan Scully: I would be very happy to tell you about the early days of quantum mechanics. I’m very interested still in these questions of non-equilibrium and thermodynamics and Bose condensation in living matter, as Fröhlich taught us to think about the problem. Let me then address your first question. What is the statistical nature of lasers, that makes it different from spontaneous emission? Fair question. Let’s go back to 1905 when Einstein used the fluctuation properties of light. He had Planck’s distribution. He understood statistical mechanics in a deep and novel way, and he started looking at fluctuation, variance in the amount of energy. He didn’t know about photons. Nobody did. They thought Maxwell gave us the right story, and that was the end of it. But then Einstein found that by looking at the entropy of this thermal radiation Planck had studied so successfully, he came to the point of realizing there are two components to the entropy of thermal light, one of which is wave-like and another of which is particle-like. He said, therefore, light has this dual nature. He came up with the photon concept. At the end of his paper, he said, “I don’t see that this violates the photoelectric effect.” It was studying fluctuations and studying the statistical properties of light way back at the beginning that led Einstein to the photon concept.
Now, fast forward 50 years, 60 years, when we are looking at problems of high intensity lasers. Glauber and others taught us that the density matrix representing such a coherent source of radiation is simple. It has a Poisson statistical distribution of photons and that’s what has often now been called the Glauber coherent state. Radio waves are a good example of Glauber coherent state, but what about the laser? It’s somewhere in between. Think about the helium neon laser, which is what we were mostly focusing on back in the 1964–1965 era. What’s the density matrix describing the radiation as it passed from below threshold, where it’s thermal, explained by Einstein, to above threshold, where it’s coherent, described by Glauber? Now, we didn’t know and Glauber gave us insight into that question.
Glauber said in his famous laser lectures concerning this question of laser radiation, let me read to you. “The only reliable method we have of constructing density operators is to devise theoretical models of the system under study and to integrate corresponding Schrödinger equation.” He says, “These assignments are formidable ones for the case of the laser oscillator and have not been carried out. It’s unlikely that we’re going to get this done.” “Nonlinearity really plays an essential role in stabilizing the field generated by lasers. It seems unlikely, therefore, that we should have a quantum mechanically consistent picture of the frequency bandwidth of the laser or of the fluctuations of its output until further progress is made with these problems.”
So, that’s Glauber speaking in his Les Houches lectures in 1964. That was the problem that Lamb had assigned me. Of course, I hadn’t seen Glauber’s statement or I would have been somewhat scared off, but I had the summer to work on it. Lamb came back and we found that, indeed, the density matrix for the laser radiation could be found using an analysis which emphasized the nonlinearity is inherent in laser behavior. I’m going to come back to that in a moment concerning the Bose condensate. But that’s the essence of what’s going on in laser radiation and how it’s different from coherent light.
Lan Yang: Among the numerous research accomplishments, which one are you most proud of?
Marlan Scully: I would turn the question around and ask, which problem did I enjoy most and was I most excited about when it worked out? Well, being a dumb graduate student working out the quantum theory of the laser, that was fun and I had Willis Lamb, talking to him every day. In the mid-80s, along comes the Bose-Einstein condensate. This is a weakly coupled gas, essentially an ideal gas, and the atoms are obeying both statistics. The wonderful researchers did the experiments, then were being summarized by Dan Kleppner at MIT. Dan is the guy who really got everybody started on this problem. It was for a long time thought by many people, including me, that it wouldn’t work. What might happen is that we just get frozen gases. Helium works, because of its Zitterbewegung effect, it’s zero-point fluctuations. But if you put a big atom in here, like rubidium, and another big atom you can polarize and stick together, I thought. Well, luckily, we were wrong and it did work. Then, Dan Kleppner wrote an article that appeared in Physics Today. He said that the Bose-Einstein condensate is really like a laser. It’s a cooperative phenomenon taking place between the atoms. This type of physics is a lot like the laser, so we’ll call it the atom laser. Oh boy. Lamb called me. He said, “Look, this is crazy. I want you to go prove that this is wrong.” He thought the way to prove it is to show that these weakly interacting atoms have essentially no nonlinearity. The atoms are not experiencing the kind of nonlinearities that photons experience, photons interacting through the presence of a gain medium, so go show that this is all wrong. And I agreed with him.
It turned out to be an interesting problem. A couple of months later I found that, gosh, they’re right. The kind of mathematics and the results that we got from the quantum theory of the optical laser, were precisely the way the equations came out after a lot of hard work. This was just taking a reasonable model and following it through in great detail. I found the same equations and I found the same density matrix that describes a laser near threshold, the helium neon laser. I was stunned and excited, so I call Lamb. I said, “Well, guess what? Kleppner is right. It is exactly an atom laser.” Willis asked for the calculations. I wrote it up and sent it to him. He was not convinced. Lamb eventually said I could publish it without his name on it. It turned out that even today people are doing experiments and finding that the fluctuations in the Bose-Einstein condensate, the atom laser, are much like they are for a photon laser. Very interesting result.
So, that now feeds into the business of biophysics and what we like to call biophotonics. Fröhlich, one of the early heroes of superconductivity. He was the first guy to point out that phonon-electron interactions were important, back in the early 50s. Then, along came Bardeen and Cooper and Schrieffer and they use this to get the BCS theory, not like Bose-Einstein. It is very interesting, however, and Fröhlich made this original early contribution. He was also saying there could be a kind of Bose condensation, a kind of coherent phenomena, in living matter. I didn’t think much about it for many years. But, within the last decade or so, our friend in Washington, DC, Dr. Bin-Salamon had stimulated this, and we’ve done the theory of the molecular protein and its longitudinal vibrational modes. It obeys the same kind of mathematics and the same results that Bose condensation and the laser obey. Looking at superfluidity and Bose condensation is an area of great excitement.
Lan Yang: What is your advice for asking great questions in research?
Marlan Scully: First pick an area that has promise, where there is a lot to be gained if you can solve the problem. I would say quantum biology is such a field. On the one hand, I could say it’s using the techniques that we have in laser spectroscopy, like FAST CARS and FASTER CARS to map out the amino acids on the surface of the COVID-19 virus. There is an example of what we might call applying quantum optical techniques to biology. On the other hand, there are deep questions, which cause us to think again. Like, Fröhlich’s question. Is there some connection between Bose condensation and lasers and living matter? In that sense, is there, perhaps, some interesting and unexpected result that we might learn by studying this problem? The answer is, likely, “yes”. The first person to really nail that question in a philosophical mode was Roger Penrose, who got the Nobel Prize this year for his pathbreaking contributions to black hole physics. He asked, is there any sense in which quantum computers might be an aspect of what’s going on in the brain? Probably not, because it’s so hot in there that you’re going to be rubbing out any coherence that’s generated.
What kind of problems should you pick? Pick a problem that interests you. Right now, I’m very interested in this question of Penrose. Can we use quantum coherence and entanglement, for example, as in superradiance, to teach us more about biology and the possibility that there could be quantum activity going on in the brain? Pick a problem that interests you. Have fun with it and stick to it.
I have to give myself negative points there. We solve problems, maybe faster than we write them up. I look back five years later and notice we never wrote that up. My advice to youngsters is don’t quit until it’s published.
Lan Yang: In addition to organizing the PQE conference, you have served on many program committees and award committees. How have you benefited from being a volunteer in the community? What is your advice to younger researchers and how can they get more involved?
Marlan Scully: I do try to help my younger colleagues and students and that’s a big thrill. Always keep an eye out for what you can do for your colleagues, because you will get a big kick out of it. See what you can nominate them for. Talk to them about what they would like and you’ll get a lot more fun out of life doing that.
Lan Yang: I will wrap up our interview with one last question. What are your hobbies outside of work? You have been referred to as “quantum cowboy.” Is there any story about that?
Marlan Scully: Maybe I was a little more aggressive when I was a young professor. People would say things like, Oh, Scully shoots first, and asks questions later. I also grew up on ranches in the backwoods of Wyoming. Hobbies were mountain climbing up Devil’s Tower and mountains in Wyoming. At Yale we had a great mountain climbing club that David Lee was part of. Fishing, ranching, these were activities that I enjoyed and still enjoy. Somehow, I got stuck with this business of being kind of a cowboy, maybe in shooting from the hip, somewhat. That’s I think how that got started. Then the fact that we have a couple working ranches probably also played into it.
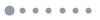
Figure 3.Cowboy physicists. Marlan Scully with Vladilen Letokhov in the late 1970s, at the Scully “ℏ” ranch in Arizona (provided by Marlan Scully).
Lan Yang: Thank you, Prof. Scully. As a well-accomplished researcher, your advice is especially insightful and useful for many of us. Thanks a lot for sharing your experiences with us.