Xuefan Yin, Chao Peng, "Manipulating light radiation from a topological perspective," Photonics Res. 8, B25 (2020)

Search by keywords or author
- Photonics Research
- Vol. 8, Issue 11, B25 (2020)
![Topological charges: topological nature of BICs and C-points [106,109,110]. (a) Schematic of radiation field decomposition for resonances of a PhC slab. The polarization vector (cx,cy) is the projection on the x−y plane of electric field ⟨uk⟩ of the radiative wave. A resonance turns into a BIC if and only if cx=cy=0. (b) Nodal lines of cx and cy. At the crossing point, polarization of resonance becomes undefined, where the BIC resides. (c) Two possible configurations of the polarization field near a BIC, giving rise to topological charges of q=+1 and q=−1. (d) Formation of half-integer charges carried by C-points split from an integer topological charge of a BIC. R(L)H, right (left) handed. (e) Measured polarization angles α in momentum space. A polarization vortex is visible in the map of α, denoted as green circles representing the location of BICs. Panels (a)–(c) are reproduced with permission from [106], copyright 2014 American Physical Society (APS); (d) is reproduced with permission from [110], copyright 2019 American Physical Society (APS); (e) is reproduced with permission from [109], copyright 2018 Springer Nature.](/richHtml/prj/2020/8/11/11000B25/img_001.jpg)
Fig. 1. Topological charges: topological nature of BICs and C-points [106,109,110]. (a) Schematic of radiation field decomposition for resonances of a PhC slab. The polarization vector ( c x , c y ) is the projection on the x − y plane of electric field ⟨ u k ⟩ of the radiative wave. A resonance turns into a BIC if and only if c x = c y = 0 . (b) Nodal lines of c x and c y . At the crossing point, polarization of resonance becomes undefined, where the BIC resides. (c) Two possible configurations of the polarization field near a BIC, giving rise to topological charges of q = + 1 and q = − 1 . (d) Formation of half-integer charges carried by C-points split from an integer topological charge of a BIC. R(L)H, right (left) handed. (e) Measured polarization angles α in momentum space. A polarization vortex is visible in the map of α , denoted as green circles representing the location of BICs. Panels (a)–(c) are reproduced with permission from [106], copyright 2014 American Physical Society (APS); (d) is reproduced with permission from [110], copyright 2019 American Physical Society (APS); (e) is reproduced with permission from [109], copyright 2018 Springer Nature.
![Bulk Fermi arc and half-integer topological charge [93]. (a) Left: Dirac points in 2D Hermitian PhC as the accidental degeneracy under C2 symmetry; middle: paired EPs in 2D non-Hermitian PhC slab spawn from Dirac points; right: examples of the isofrequency contours, including the open bulk Fermi arc connecting two EPs (middle panel), and closed contours at higher (upper panel) or lower (lower panel) frequencies. (b) Numerically simulated and experimentally measured isofrequency contours at three representative wavelengths. The bulk Fermi arc appears at 791.0 nm (middle panels), when the isofrequency contour becomes open-ended. (c) Experimental demonstration of the half-integer topological charges around the bulk Fermi arc. Left: schematic of polarization winding along a loop enclosing an EP; middle and right: experimental and simulated results of the polarization information along the isofrequency contour, exhibiting a half-integer topological charge of polarization vectors. Panels (a)–(c) are reproduced with permission from [93], copyright 2018 American Association for the Advancement of Science (AAAS).](/richHtml/prj/2020/8/11/11000B25/img_002.jpg)
Fig. 2. Bulk Fermi arc and half-integer topological charge [93]. (a) Left: Dirac points in 2D Hermitian PhC as the accidental degeneracy under C 2 symmetry; middle: paired EPs in 2D non-Hermitian PhC slab spawn from Dirac points; right: examples of the isofrequency contours, including the open bulk Fermi arc connecting two EPs (middle panel), and closed contours at higher (upper panel) or lower (lower panel) frequencies. (b) Numerically simulated and experimentally measured isofrequency contours at three representative wavelengths. The bulk Fermi arc appears at 791.0 nm (middle panels), when the isofrequency contour becomes open-ended. (c) Experimental demonstration of the half-integer topological charges around the bulk Fermi arc. Left: schematic of polarization winding along a loop enclosing an EP; middle and right: experimental and simulated results of the polarization information along the isofrequency contour, exhibiting a half-integer topological charge of polarization vectors. Panels (a)–(c) are reproduced with permission from [93], copyright 2018 American Association for the Advancement of Science (AAAS).
![Manipulation radiation characteristics from topological charges [124,128]. (a) Topological charges evolution for merging BICs. Middle panel represents the configuration of merging BICs. (b) Scaling rules of Q factors along with k-points for isolated BICs and merging BICs. (c) Experimentally measured Q factors for merging BICs (left) and isolated BICs (right) under the same fabrication process. (d) Topological charge evolution for UGRs. Charges with q=±1/2 in the middle panel denote the C-points corresponding to CP states with opposite helicity. Right panel represents the configuration of UGRs. (e) Profile of electric field component Ey of UGR. (f) Experimental and simulated results of downward Q factors around UGR. (g) Experimentally measured trajectories of two half-integer topological charges that evolve along with the varied width of air bars of 1D PhC slab w. The crossing point of two trajectories verifies the existence of UGR. Panels (a)–(c) are reproduced with permission from [124], copyright 2019 Springer Nature; panels (d)–(g) are reproduced with permission from [128], copyright 2020 Springer Nature.](/Images/icon/loading.gif)
Fig. 3. Manipulation radiation characteristics from topological charges [124,128]. (a) Topological charges evolution for merging BICs. Middle panel represents the configuration of merging BICs. (b) Scaling rules of Q factors along with k -points for isolated BICs and merging BICs. (c) Experimentally measured Q factors for merging BICs (left) and isolated BICs (right) under the same fabrication process. (d) Topological charge evolution for UGRs. Charges with q = ± 1 / 2 in the middle panel denote the C-points corresponding to CP states with opposite helicity. Right panel represents the configuration of UGRs. (e) Profile of electric field component E y of UGR. (f) Experimental and simulated results of downward Q factors around UGR. (g) Experimentally measured trajectories of two half-integer topological charges that evolve along with the varied width of air bars of 1D PhC slab w . The crossing point of two trajectories verifies the existence of UGR. Panels (a)–(c) are reproduced with permission from [124], copyright 2019 Springer Nature; panels (d)–(g) are reproduced with permission from [128], copyright 2020 Springer Nature.
![Generate vortex beams based on topological charges [133,134]. (a) Top: schematic illustration of the designed perovskite metasurface pumped by laser light, generating a vortex beam based on topological charge; bottom: donut-shaped emission profile and inverted fork-shaped self-interference pattern of output vortex beam. (b) Dynamic control of output beam based on manipulating the optical gain profile. Schematics of the experiments (top) and experimentally measured emission patterns (bottom). The emission profile switches to two lobes (right panels) from the donut shape (left panels) when the profile of the pumping light is changed from a circle to an ellipse. (c) Top: transition from a donut beam to a linearly polarized two-lobe beam. Insets are corresponding emission profiles of the output beam; bottom: transition from a donut beam to a two-lobe beam and back, within a picosecond-scale transition time. (d) Schematic illustration of a passive vortex beam generator based on a PhC slab. (e) Far-field patterns and interferograms of output beams under different incident polarization. (f) Polarization winding of topological charge q=−2. (g) Vortex beam with OAM of l=4 based on topological charge of q=−2. Panels (a)–(c) are reproduced with permission from [133], copyright 2020 AAAS; panels (d)–(g) are reproduced with permission from [134], copyright 2020 Springer Nature.](/Images/icon/loading.gif)
Fig. 4. Generate vortex beams based on topological charges [133,134]. (a) Top: schematic illustration of the designed perovskite metasurface pumped by laser light, generating a vortex beam based on topological charge; bottom: donut-shaped emission profile and inverted fork-shaped self-interference pattern of output vortex beam. (b) Dynamic control of output beam based on manipulating the optical gain profile. Schematics of the experiments (top) and experimentally measured emission patterns (bottom). The emission profile switches to two lobes (right panels) from the donut shape (left panels) when the profile of the pumping light is changed from a circle to an ellipse. (c) Top: transition from a donut beam to a linearly polarized two-lobe beam. Insets are corresponding emission profiles of the output beam; bottom: transition from a donut beam to a two-lobe beam and back, within a picosecond-scale transition time. (d) Schematic illustration of a passive vortex beam generator based on a PhC slab. (e) Far-field patterns and interferograms of output beams under different incident polarization. (f) Polarization winding of topological charge q = − 2 . (g) Vortex beam with OAM of l = 4 based on topological charge of q = − 2 . Panels (a)–(c) are reproduced with permission from [133], copyright 2020 AAAS; panels (d)–(g) are reproduced with permission from [134], copyright 2020 Springer Nature.
![Generate vortex beams based on spin-momentum locking [135,137]. (a) Top: scanning electron microscope (SEM) image of a topological vortex laser with an X-shaped PhC cavity (red lines). The corresponding spin direction of the cavity mode is indicated by the arrows. Bottom: zoomed-in SEM image of the topological interface. Red line, topological interface; blue hexagon, unit cell in the topological PhC; orange hexagon, unit cell in the trivial PhC. (b) Schematic illustration of angular-momentum conservation of the CP vortex beam with respect to the spin-momentum-locking edge states. (c) Energy band structure of the discrete edge states (red and green dots) with surrounding bulk bands (gray lines). Red dots, spin-up edge states; green dots, spin-down edge states. (d) Pattern of self-interference of the output beam, verifying OAM of l=−2. (e) SEM images of the vortex beam generator based on WGMs in the ring resonator. (f) Non-Hermitian interactions and corresponding emission pattern. Top panels: without non-Hermitian interactions, no phase winding exists in the output beam; middle and bottom panels: with unidirectional non-Hermitian interaction, the self-interference pattern exhibits an inverted fork shape, verifying the vortex beam. (g) Five channels of different OAMs are realized in microlasers shown in (e). Panels (a)–(c) are reproduced with permission from [133], copyright 2020 American Physical Society (APS); panels (d)–(g) are reproduced with permission from [134], copyright 2020 AAAS.](/Images/icon/loading.gif)
Fig. 5. Generate vortex beams based on spin-momentum locking [135,137]. (a) Top: scanning electron microscope (SEM) image of a topological vortex laser with an X -shaped PhC cavity (red lines). The corresponding spin direction of the cavity mode is indicated by the arrows. Bottom: zoomed-in SEM image of the topological interface. Red line, topological interface; blue hexagon, unit cell in the topological PhC; orange hexagon, unit cell in the trivial PhC. (b) Schematic illustration of angular-momentum conservation of the CP vortex beam with respect to the spin-momentum-locking edge states. (c) Energy band structure of the discrete edge states (red and green dots) with surrounding bulk bands (gray lines). Red dots, spin-up edge states; green dots, spin-down edge states. (d) Pattern of self-interference of the output beam, verifying OAM of l = − 2 . (e) SEM images of the vortex beam generator based on WGMs in the ring resonator. (f) Non-Hermitian interactions and corresponding emission pattern. Top panels: without non-Hermitian interactions, no phase winding exists in the output beam; middle and bottom panels: with unidirectional non-Hermitian interaction, the self-interference pattern exhibits an inverted fork shape, verifying the vortex beam. (g) Five channels of different OAMs are realized in microlasers shown in (e). Panels (a)–(c) are reproduced with permission from [133], copyright 2020 American Physical Society (APS); panels (d)–(g) are reproduced with permission from [134], copyright 2020 AAAS.
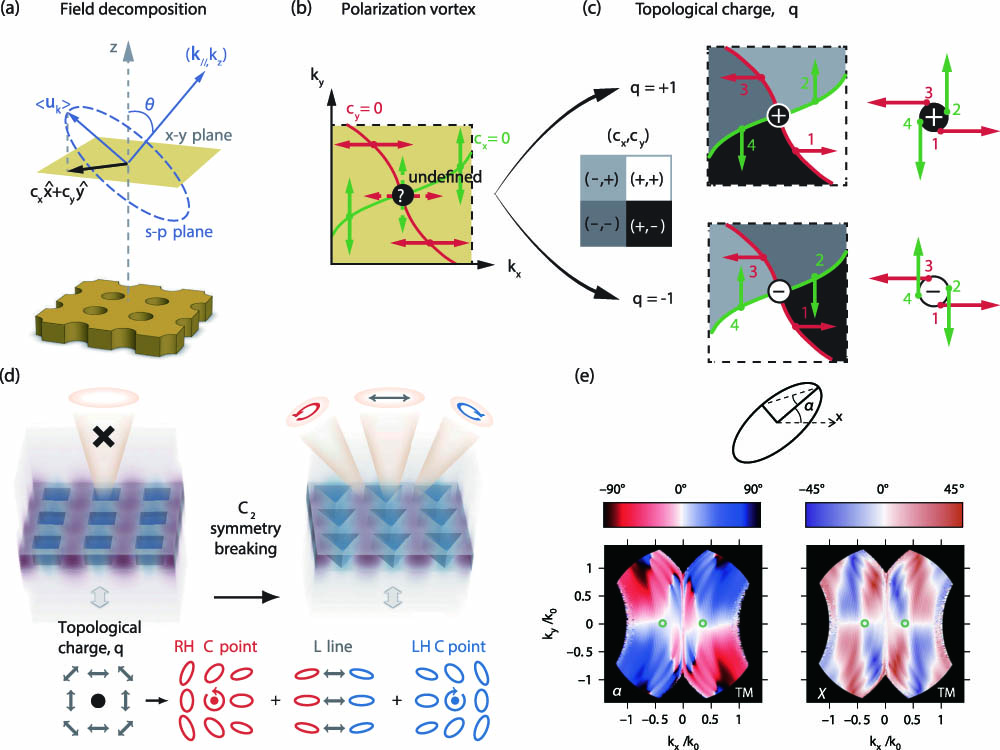
Set citation alerts for the article
Please enter your email address