P. Boucaud, M. El Kurdi, A. Ghrib, M. Prost, M. de Kersauson, S. Sauvage, F. Aniel, X. Checoury, G. Beaudoin, L. Largeau, I. Sagnes, G. Ndong, M. Chaigneau, R. Ossikovski, "Recent advances in germanium emission [Invited]," Photonics Res. 1, 102 (2013)

Search by keywords or author
- Photonics Research
- Vol. 1, Issue 3, 102 (2013)
![Calculated dependence of photoinduced carrier density needed to reach transparency at room temperature in n-doped germanium as a function of tensile strain. The germanium doping is 2×1019 cm−3. The calculation is based on the 30-band k·p formalism that we presented in [16]. The squares account for free-carrier absorption, as reported in [13]. The circles correspond to a calculation that considers the experimental absorption cross section for electrons and holes reported in [20] and a power law dependence as a function of wavelength with an exponent of 2.25 for electrons and 2.43 for holes.](/richHtml/prj/2013/1/3/03000102/img_001.jpg)
Fig. 1. Calculated dependence of photoinduced carrier density needed to reach transparency at room temperature in n -doped germanium as a function of tensile strain. The germanium doping is 2 × 10 19 cm − 3 . The calculation is based on the 30-band k · p formalism that we presented in [16]. The squares account for free-carrier absorption, as reported in [13]. The circles correspond to a calculation that considers the experimental absorption cross section for electrons and holes reported in [20] and a power law dependence as a function of wavelength with an exponent of 2.25 for electrons and 2.43 for holes.
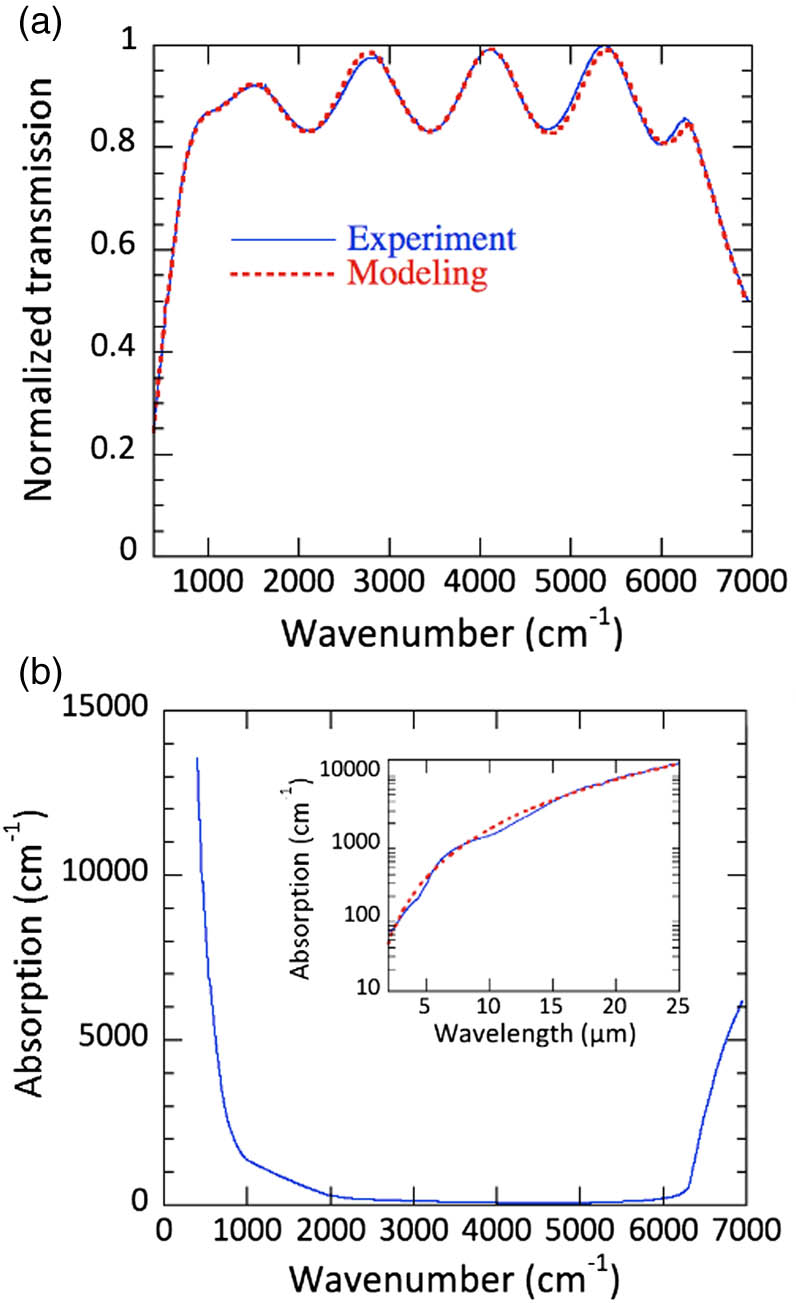
Fig. 2. (a) Transmission of a Ge/GaAs film normalized by the transmission of a GaAs wafer. Both wafers are double-side polished. The germanium thickness is 930 nm. The doping of the germanium layer is 10 19 cm − 3 . The germanium absorption is deduced from this normalized transmission. The dashed line corresponds to the modeling of the transmission that accounts for the germanium absorption. (b) Absorption of the germanium film as a function of wavenumber. The inset shows a zoom of the absorption in the 2–25 μm spectral range. The dashed line corresponds to a power fit with a 2.25 exponent (absorption ∝ λ 2.25 ).
![(a) Scanning electron microscopy image of tensile-strained germanium microdisk. (b) Room temperature photoluminescence spectrum of an unstrained (bottom) and strained (top) germanium microdisk with a 4 μm diameter. (c) Enlargement of the emission around 2000 nm [48].](/Images/icon/loading.gif)
Fig. 3. (a) Scanning electron microscopy image of tensile-strained germanium microdisk. (b) Room temperature photoluminescence spectrum of an unstrained (bottom) and strained (top) germanium microdisk with a 4 μm diameter. (c) Enlargement of the emission around 2000 nm [48].
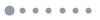
Fig. 4. (a) In-plane strain map of the germanium microdisk as deduced from backscattering μ -Raman spectroscopy. (b) Strain profile along the disk diameter. The disk diameter is 9 μm. The figure highlights the sum of the in-plane strain components. In Fig. 1 , only one component is considered to describe the strain.
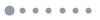
Fig. 5. (a) Conduction band profile of a GaAs/Ge/GaAs heterostructure without applied bias. (b) Conduction band profile for a 1.5 V applied bias. The quasi-Fermi levels are indicated by dashed lines.
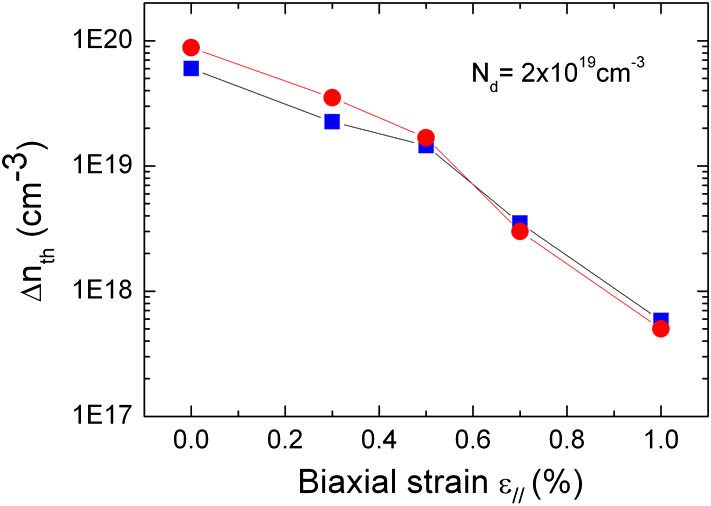
Set citation alerts for the article
Please enter your email address