Xixi Chen, Tianli Wu, Zhiyong Gong, Yuchao Li, Yao Zhang, Baojun Li, "Subwavelength imaging and detection using adjustable and movable droplet microlenses," Photonics Res. 8, 225 (2020)

Search by keywords or author
- Photonics Research
- Vol. 8, Issue 3, 225 (2020)
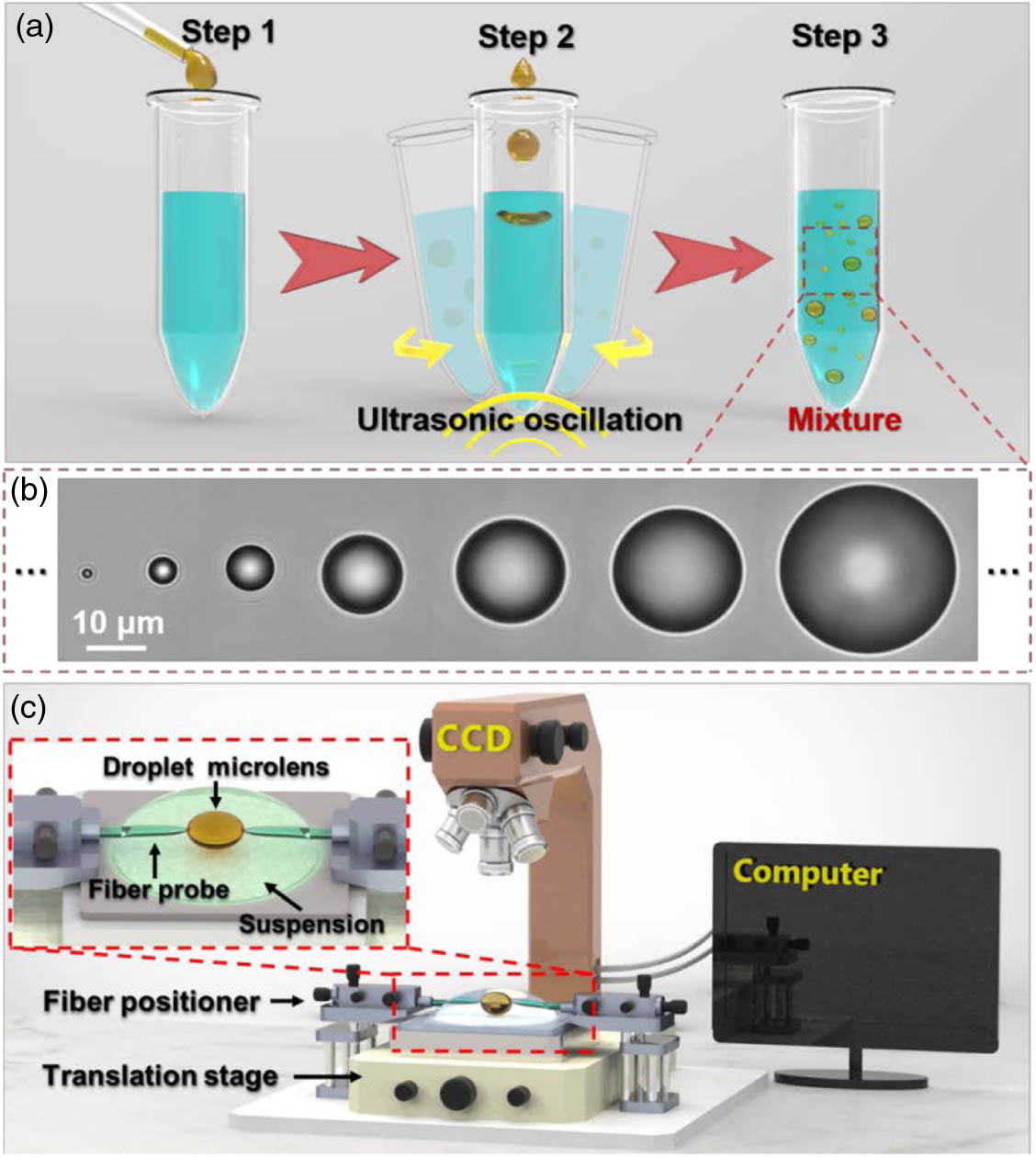
Fig. 1. Droplet preparation and experimental setup. (a) Preparation process of the droplet microlenses. (b) Optical microscope images of the droplet microlenses showing they are spheroids of different sizes. (c) Schematic of the experimental setup. The inset shows a schematic of the microlens deformation.
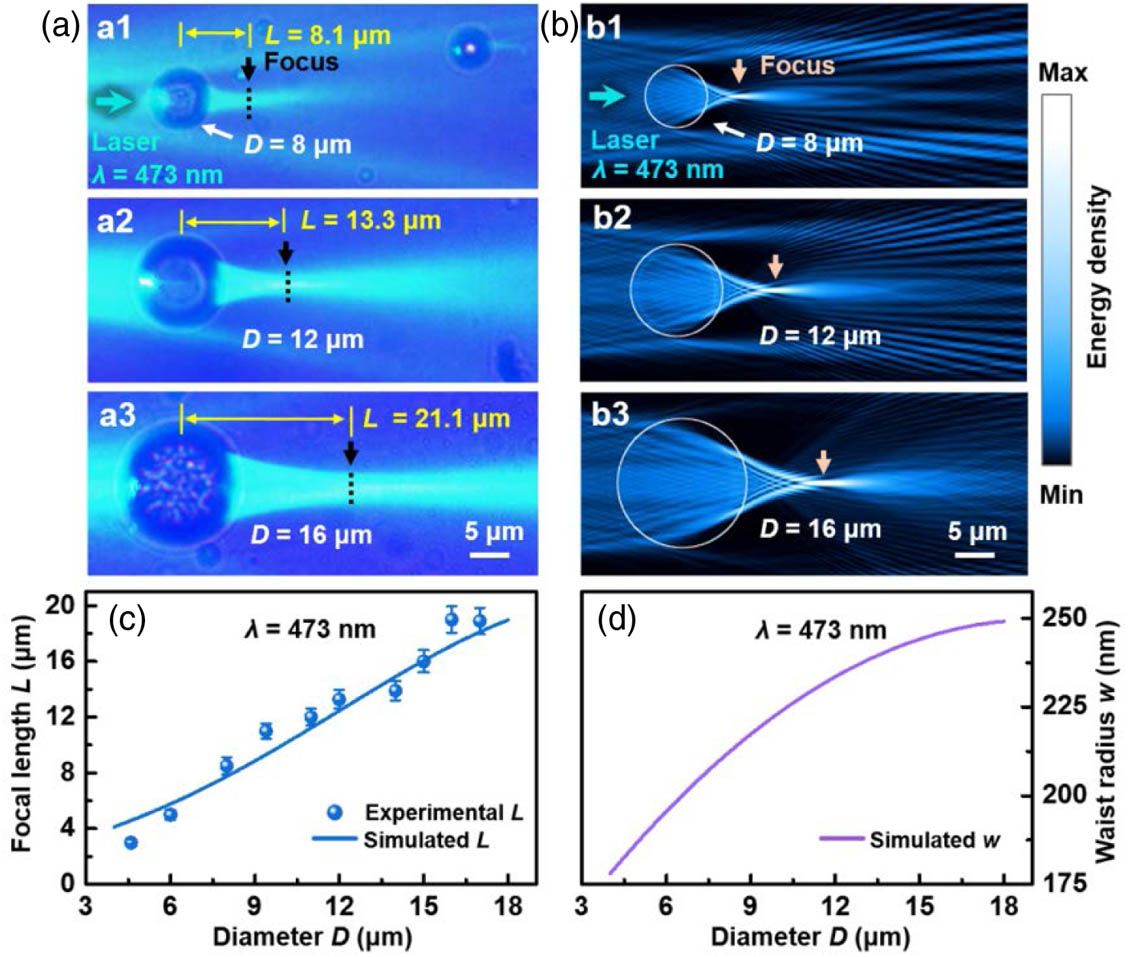
Fig. 2. Focusing of the droplet microlenses in different sizes. (a) Optical microscope images of microlenses with diameters D of (a1) 8, (a2) 12, and (a3) 16 μm. (b) Simulated energy density distribution for each microlens. (c) L as a function of D at a fixed wavelength of 473 nm. (d) Waist radius (w ) as a function of D at a fixed wavelength of 473 nm.
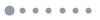
Fig. 3. Focusing of the droplet microlenses of different shapes. (a) Optical microscope images of microlenses with shapes of (a1) a sphere with a diameter D of 12 μm, (a2) an ellipsoid with a ratio between the semimajor and semiminor axes (A / B ) of 1.3, and (a3) an ellipsoid with A / B = 1.7 . (b) Simulated energy density distribution for each microlens. (c) Focal length L and waist radius (w ) of the output beams as functions of A / B at a fixed wavelength of 473 nm.
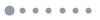
Fig. 5. Subwavelength imaging. (a) Schematic of subwavelength imaging with the droplet microlens. (b) SEM image of the gratings of a commercial Blu-ray Disk (BD). Optical microscope images of the BD gratings with the assistance of droplet microlenses with diameters of (c) 4.8, (d) 7.7, and (e) 13.6 μm. (f) SEM image of the stack of PS nanoparticle layers formed by evaporation-induced assembly. Inset is a magnified view of the PS nanoparticles. (g) Size distribution of the PS nanoparticles. (h) Optical microscope image of the PS nanoparticles obtained with the assistance of a 2 × 2 microlens array. The inset shows the magnified imaging of the view field of the droplet microlens. (i) Intensity variation of (h) along the transverse cross section through the center of two light spots (α and β ) from the PS nanoparticles (D = 80 nm ).
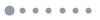
Fig. 6. Signal enhancement. (a) Optical microscope images of QD fluorescence enhancement. (a1) A QD cluster was located on the SiO 2 slide, and then microlenses with diameters D of (a2) 11.8, (a3) 9.2, and (a4) 6.8 μm were separately moved right above the QD cluster. (b) Fluorescence images of the QD cluster captured (b1) without and (b2)–(b4) with the assistance of microlenses. (c) Profiles of the intensity distributions corresponding to the dark-field images. (d) Illustration of the model used to calculate the effective numerical aperture NA eff . (e) Schematic of the enhancement of Raman scattering signals from a silicon (Si) wafer. (f) Intensities of Raman scattering from the Si wafer without and with the assistance of the droplet microlenses with D of 45, 21, and 8 μm.
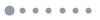
Fig. 7. Transmittance spectrum of 1-bromonaphthalene (C 10 H 7 Br ) with the wavelength range from 300 to 900 nm.
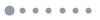
Fig. 8. The diameter distribution of the droplet at f = 80 kHz and t = 5 min .
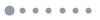
Fig. 9. Fluctuation region of Brownian motion of trapped microlens (D = 1 μm ) as a function of trapping power.
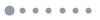
Fig. 10. Droplet microlens diameter versus intensity of Raman scattering signals. The fitting method is based on a polynomial fit.
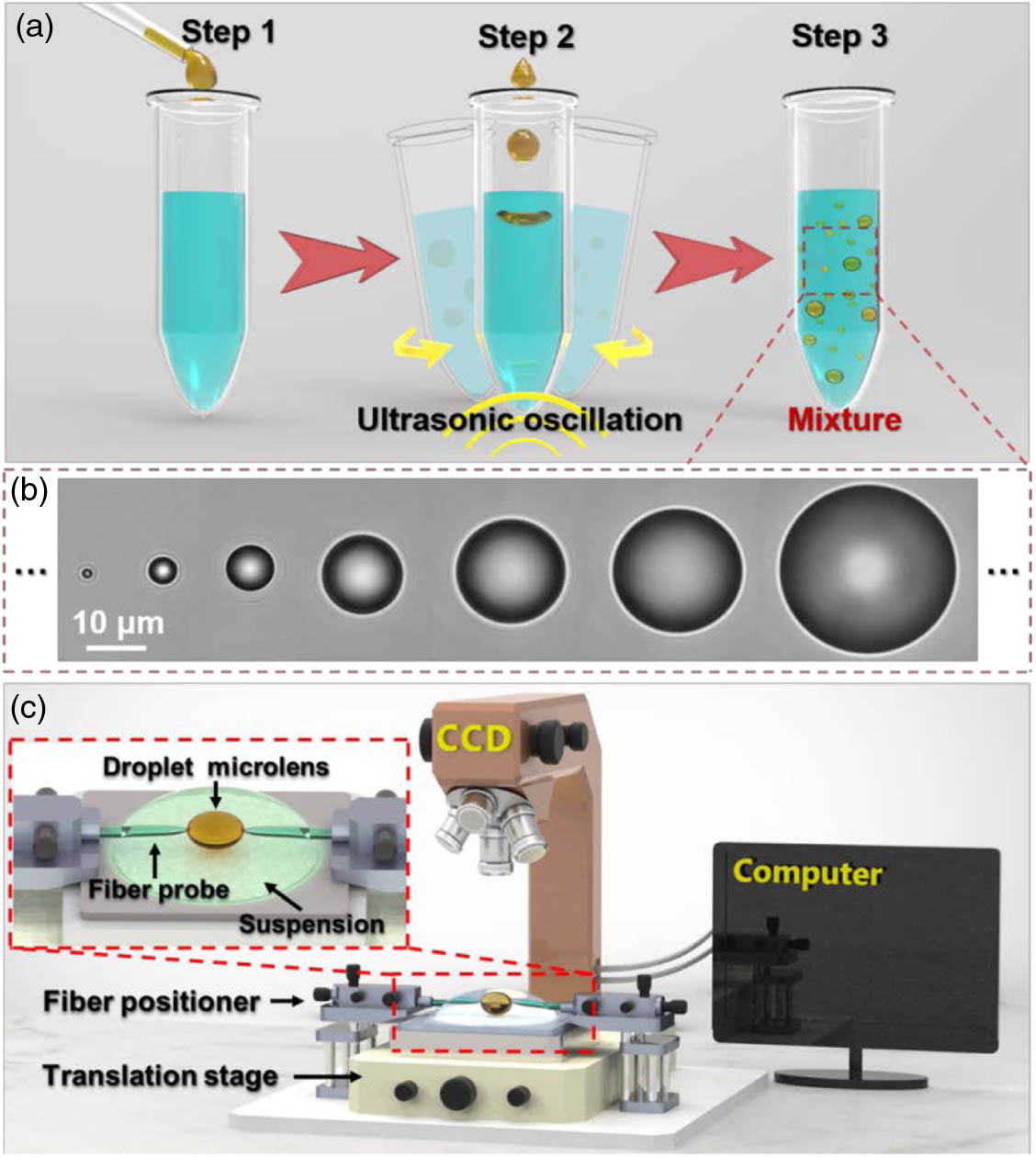
Set citation alerts for the article
Please enter your email address