Author Affiliations
1Shenzhen Key Laboratory of Ultraintense Laser and Advanced Material Technology, Center for Advanced Material Diagnostic Technology, College of Engineering Physics, Shenzhen Technology University, Shenzhen , Guangdong 518118, China2College of Physics and Optoelectronic Engineer, Shenzhen University, Shenzhen , Guangdong 518060, Chinashow less
Abstract
A diode pumped high energy Yb∶YAG rod regenerative amplifier was demonstrated with a maximum energy of 22.3 mJ, excellent energy stability (~0.8% root mean square), and beam quality (M2 < 1.2) at 10 Hz repetition rate. To the best of our knowledge, this is the highest energy so far obtained by a Yb∶YAG rod regenerative amplifier.1 Introduction
Ultraintense ultrashort lasers are widely used in strong-field physics,such as high harmonic generation[1-2],nonlinear-optical processes[3],and multicolor pulse synthesizers[4],to compact coherent X-ray sources based on inverse Compton scattering[5]. At present,these lasers are used in Ti∶sapphire-based chirped pulse amplification(CPA)[6-8] or nonlinear crystal-based optical parametric CPA(OPCPA)[9-10] devices. However,such devices require high-quality nanosecond green lasers for pumping;thus,they are bulky,expensive,and inefficient,which limits their large-scale applications in laboratories and industries.
Yb∶YAG gain-medium-based lasers have rapidly gained prominence in the past two decades due to their excellent spectral,thermal,and mechanical characteristics,such as long storage lifetime,large emission cross-section [11],broad emission bandwidth [12],low quantum defect,high thermal conductivity [13],and large Mohs hardness. In 2016,based on a thin-disk Yb∶YAG laser,Jung et al.[14] realized 300 mJ energy with a 2 ps pulse duration at 100 Hz. Nubbemeyer et al.[15] increased the average power to 1 kW,and the pulse energy reached 200 mJ with a 1.1 ps pulse duration. Based on an Innoslab Yb∶YAG laser,in 2011,Schulz et al.[16] obtained parameters of 20 mJ,830 fs,and 12.5 kHz. These studies have shown extremely high laser parameters. However,the thin-disk and Innoslab structures experience inherent problems,e.g.,challenging fabrication,complicated beam-shaping,and high maintenance costs.
A repetition frequency of 10 Hz can meet requirements for many strong-field physical experiments[17-18]. In this study,a diode-pumped Yb∶YAG rod regenerative amplifier combined with nonlinear compression to achieve a terawatt(TW)peak power laser pulse is proposed to reduce the cost of ultraintense ultrashort lasers. As shown in Fig. 1,the seed is broadband and high energy from a solid-state oscillator or fiber oscillator plus fiber preamplifier;it is injected into a stretcher with a nanosecond output pulse duration. To achieve spectral shaping,we need to drill holes for the center wavelength to prevent the gain narrowing effect during the amplification phase. It is then seeded into a Yb∶YAG rod-based regenerative amplifier with more than 100 mJ output energy. Two stages of compression are applied after amplification. The first stage involves a conventional Treacy-type compressor that compresses the pulse duration to less than 1 ps with an efficiency of approximately 70%. The second stage involves a multipass Herriot-type noble-gas-filled cell [19-20],which compresses the pulse to approximately 50 fs with an efficiency of more than 70% and compress ratio of more than 20. As a result,a simple,stable,and low-cost TW-class ultraintense ultrashort laser is obtained.
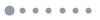
Figure 1.Conceptual design of Yb∶YAG rod amplifier and nonlinear-compression-based TW laser system
In this study,a high energy,diode-pumped Yb∶YAG rod regenerative amplifier is demonstrated. The nanosecond laser output energy was as high as 22.3 mJ,and the root-mean-square(RMS)energy variation was as small as 0.8%,with M2 < 1.2 near the diffraction-limited beam. To the best of our knowledge,this is the highest energy ever obtained by a Yb∶YAG rod regenerative amplifier.
2 Experimental setup
Fig. 2 illustrates the layout of the diode-pumped Yb∶YAG rod regenerative amplifier. The seed was a single longitudinal mode fiber laser with a full width at half-maximum(FWHM)pulse of 4 ns and pulse energy of less than 1 nJ. The Yb∶YAG rod(5 mm×5 mm×10 mm,2% doped)was connected to a Cu heat sink with indium foil over its lateral area. The pump is a fiber-coupled diode laser(BWT Inc.,China)with 200 W maximum peak power and 135 μm inner core diameter centered at 940 nm. A digital delay signal generator(SRS,DG645)was used to control the trigger and delay between the seed,pump,and Pockels cell(PC).
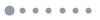
Figure 2.Experimental setup
In terms of cavity design,the length of the resonant cavity was 2.2 m. The round-trip time of photons in the cavity was 14.7 ns. The laser rod was the symmetric center of the cavity. On each side of the crystal are two large-curvature-radius concave mirrors(right-side is M1 and M3;left-side is M4 and M6)and one large-curvature-radius convex mirror(right-side is M2;left-side is M5),making the beam-radius variation less than 3.4% within the range of -200 mm to 200 mm from the symmetry center of the cavity. To avoid optical damage,the cavity was designed with a 1 mm mode diameter and a 1.9 mm PC side diameter,having a low damage threshold. The mode diameter was optimized for maximum output energy with a pump-to-mode diameter ratio of 0.8. To control the amplification passes,a quarter-wave plate(QW),thin-film polarizer(TFP),and PC were used for optical switching. To separate the input and output beams,a Faraday rotator(FR),half-wave plate(HW),and TFP were employed.
3 Results
The system was first characterized in a free-running(continuous-wave)condition,and then the seed was injected into the regenerative amplifier.
3.1 Free-running operation
The regenerative cavity was adjusted and optimized under a free-running condition with quasicontinuous wave(QCW)pumping(10 Hz,Fig. 3). In this case,the PC was switched off,and the QW and TFP formed an output coupling mirror. The experimentally measured Yb∶YAG rod absorption efficiency of the pump was ~75%. A maximum slope efficiency of 36% could be attained with a 1 ms pump pulse width. When the pump pulse widths were 1.5 ms and 2 ms,the threshold increased,and the maximum output energy drastically increased. The slope efficiency slightly decreased but was still above 30%,and there was no saturation trend. The inset in Fig. 3 shows a well-formed near-field beam profile of the output laser with a 1.5 ms pump pulse width and 225 mJ absorbed pump energy.
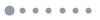
Figure 3.Output energy as a function of absorbed pump energy for free running (inset: near-field beam profile with 225 mJ absorbed pump energy and 1.5 ms pump pulse width)
3.2 Injected seed operation
With the optimized cavity,the seed was injected,and the PC was enabled. Fig. 4(a)shows the round-trip time and output energy with a 1.5 ms pump pulse width at 10 Hz. With an increase in absorbed pump energy,the round-trip time(i.e.,amplification passes)decreased and the output energy increased. With absorbed pump energy of more than 180 mJ,the output energy tended to be saturated. At the maximum absorbed pump energy of 225 mJ,the seed was amplified to 22.3 mJ. The intracavity pulse-amplification evolution was measured using a photodiode and oscilloscope;the results are shown in Fig. 4(b). In the nonsaturated amplification stage,the round-trip gain was ~2. Once saturation was reached,the laser switched out.
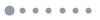
Figure 4.Results. (a) Round-trip time and output energy with 1.5 ms pump pulse width at 10 Hz; (b) intracavity pulse-amplification evolution
Fig. 5(a)shows the temporal shape of the switched-out laser pulse. The pulse waveform was clean without pre- and post-pulses. Fig. 5(b)shows the laser spectrum. The center wavelength was 1030 nm at a 0.8 nm bandwidth(FWHM)in the Q-switched(without seed)condition. Therefore,if the input seed is an ns-level broadband chirped pulse,a bandwidth of at least 0.8 nm after amplification could be maintained,and the gain could be maintained simultaneously.
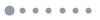
Figure 5.Pulse waveform. (a) Temporal pulse shape of regenerated output; (b) input seed spectrum, Q-switched spectrum, and amplified-pulse spectrum
Fig. 6(a)shows the measured beam-propagation parameter at 20 mJ output energy. The fitted M2 values were 1.17 and 1.18 in the horizontal and vertical directions,respectively. The inset is the far-field beam profile. Fig. 6(b)shows the stability of the experimental output energy under a 2 h test. The RMS variability was as small as 0.8%.
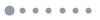
Figure 6.Results. (a) M2 factor of output laser beam (inset: far-field beam profile); (b) 2 h output-energy stability test result
4 Conclusion
In summary,a diode-pumped Yb∶YAG rod regenerative amplifier was developed. Owing to a QCW pump and progressive cavity design,the regenerative amplifier boosted the seed from nanojoules to 22.3 mJ with high energy stability(RMS variability <0.8%)and obtained both a near-diffraction-limited beam(M2 <1.2)and 0.8 nm Q-switched spectrum bandwidth. Future works include enlarging the cavity-mode diameter,increasing the pump power,and injecting a broadband chirped seed,thereby increasing the energy to 50 mJ with a 0.8 nm chirped pulse. Afterward,a cascaded two-stage compression would be performed;thus,a low-cost TW-class laser is realizable.
References
[1] Constant E, Garzella D, Breger P et al. Optimizing high harmonic generation in absorbing gases: model and experiment[J]. Physical Review Letters, 82, 1668-1671(1999).
[2] Boltaev G S, Ganeev R A, Kim V V et al. High-order harmonics generation in the plasmas produced on different rotating targets during ablation using 1 kHz and 100 kHz lasers[J]. Optics Express, 28, 18859-18875(2020).
[3] Zhu Y, Elim H I, Foo Y L et al. Multiwalled carbon nanotubes beaded with ZnO nanoparticles for ultrafast nonlinear optical switching[J]. Advanced Materials, 18, 587-592(2006).
[4] Huang S W, Cirmi G, Moses J et al. High-energy pulse synthesis with sub-cycle waveform control for strong-field physics[J]. Nature Photonics, 5, 475-479(2011).
[5] Graves W S, Bessuille J, Brown P et al. Compact X-ray source based on burst-mode inverse Compton scattering at 100 kHz[J]. Physical Review Special Topics-Accelerators and Beams, 17, 120701(2014).
[6] Dubietis A, Jonušauskas G, Piskarskas A. Powerful femtosecond pulse generation by chirped and stretched pulse parametric amplification in BBO crystal[J]. Optics Communications, 88, 437-440(1992).
[7] Guo X Y, Xu Y, Zou X et al. Non-collinear phase-matching geometries in optical parametric chirped-pulse amplification[J]. Optics Communications, 330, 24-29(2014).
[8] Zeng X M, Zhou K N, Zuo Y L et al. Multi-petawatt laser facility fully based on optical parametric chirped-pulse amplification[J]. Optics Letters, 42, 2014-2017(2017).
[9] Hrisafov S, Pupeikis J, Chevreuil P A et al. High-power few-cycle near-infrared OPCPA for soft X-ray generation at 100 kHz[J]. Optics Express, 28, 40145-40154(2020).
[10] Batysta F, Antipenkov R, Novák J et al. Broadband OPCPA system with 11 mJ output at 1 kHz, compressible to 12 fs[J]. Optics Express, 24, 17843-17848(2016).
[11] Ye P P, Zhu S Q, Li Z et al. Passively Q-switched dual-wavelength green laser with an Yb∶YAG/Cr4+ composite crystal[J]. Optics Express, 25, 5179-5185(2017).
[12] Jornod N, Wittwer V J, Kränkel C et al. High-power amplification of a femtosecond vertical external-cavity surface-emitting laser in an Yb∶YAG waveguide[J]. Optics Express, 25, 16527-16533(2017).
[13] Divoky M, Pilar J, Hanus M et al. Performance comparison of Yb∶YAG ceramics and crystal gain material in a large-area, high-energy, high average-power diode-pumped laser[J]. Optics Express, 28, 3636-3646(2020).
[14] Jung R, Tümmler J, Will I. Regenerative thin-disk amplifier for 300 mJ pulse energy[J]. Optics Express, 24, 883-887(2016).
[15] Nubbemeyer T, Kaumanns M, Ueffing M et al. 1 kW, 200 mJ picosecond thin-disk laser system[J]. Optics Letters, 42, 1381-1384(2017).
[16] Schulz M, Riedel R, Willner A et al. Yb∶YAG innoslab amplifier: efficient high repetition rate subpicosecond pumping system for optical parametric chirped pulse amplification[J]. Optics Letters, 36, 2456-2458(2011).
[17] Serrat C, Roca D, Budesca J M et al. Avalanche of stimulated forward scattering in high harmonic generation[J]. Optics Express, 24, 8028-8044(2016).
[18] Gazibegović-Busuladžić A, Becker W, Milošević D B. Helicity asymmetry in strong-field ionization of atoms by a bicircular laser field[J]. Optics Express, 26, 12684-12697(2018).
[19] Schulte J, Sartorius T, Weitenberg J et al. Nonlinear pulse compression in a multi-pass cell[J]. Optics Letters, 41, 4511-4514(2016).
[20] Kaumanns M, Pervak V, Kormin D et al. Multipass spectral broadening of 18 mJ pulses compressible from 1.3 ps to 41 fs[J]. Optics Letters, 43, 5877-5880(2018).