Xiaojiong Chen, Zhaorong Fu, Qihuang Gong, Jianwei Wang, "Quantum entanglement on photonic chips: a review," Adv. Photon. 3, 064002 (2021)

Search by keywords or author
- Advanced Photonics
- Vol. 3, Issue 6, 064002 (2021)
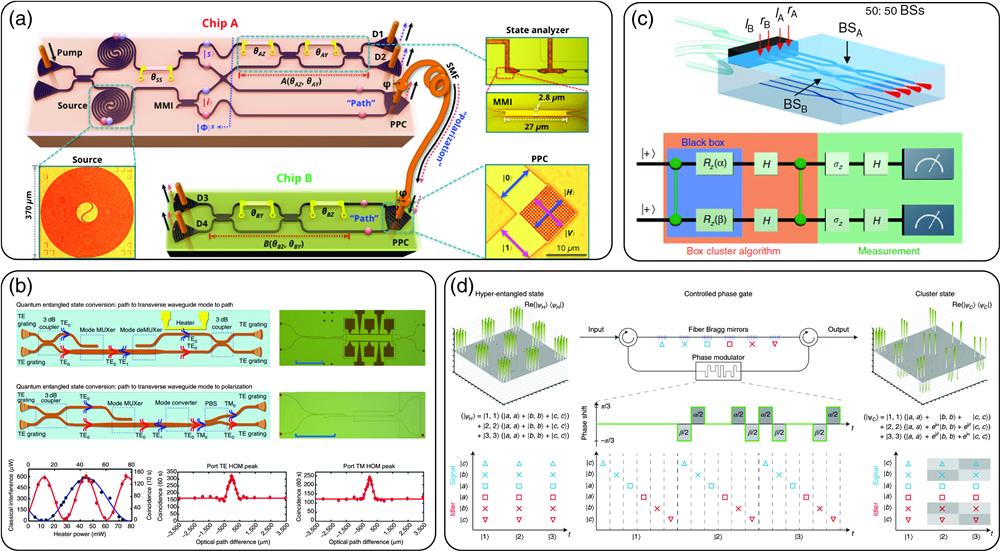
Fig. 1. On-chip conversion of multiple DoFs and multi-DoF entanglement. (a) Chip-to-chip quantum entanglement distribution by path-polarization interconversion. A pair of entangled photons, coded in path, were generated on chip A, then one photon was distributed to chip B via two-dimensional grating couplers, while the other photon remained on chip A. As a result, a two-photon entangled state was distributed coherently between two separate chips. Bell-type violation of showed the strong entanglement between two separate photons. (b) Quantum entangled state conversion between different DoFs of path, transverse mode, and polarization. Two photons were coupled into the silicon chip, and then NOON entangled state, coded in path, was generated by an interference. With a mode multiplexer, the entangled state coded in path was first converted into a transverse waveguide mode . Then, the quantum information was converted back to the path by a mode demultiplexer or to polarization by a PBS. Two-photon interference and Hong–Ou–Mandel effect measurements show the preservation of quantum coherence during the conversion between different DoFs. (c) Four-qubit hyperentanglement cluster state by entangling the path and polarization simultaneously. Quantum state tomography and genuine multipartite entanglement witness were analyzed to ensure high fidelity of the prepared four-qubit cluster state. Based on the model of one-way quantum computing, Grover’s search algorithm was performed, where the average success rate of the algorithm was . (d) Two photon 4-qutrit hyperentangled cluster state. Three-level time-bin entangled state with three frequency modes was created on a micro-ring resonator. Then, hyperentanglement of time-frequency was realized by a controlled phase gate (composed of fiber Bragg mirrors and an electro-optical phase modulator). Basic high-dimensional one way quantum computing operators were tested. Panels reproduced from: (a) Ref. 67, Optica; (b) Ref. 68, Springer Nature; (c) Ref. 34, Springer Nature; and (d) Ref. 72, Springer Nature.
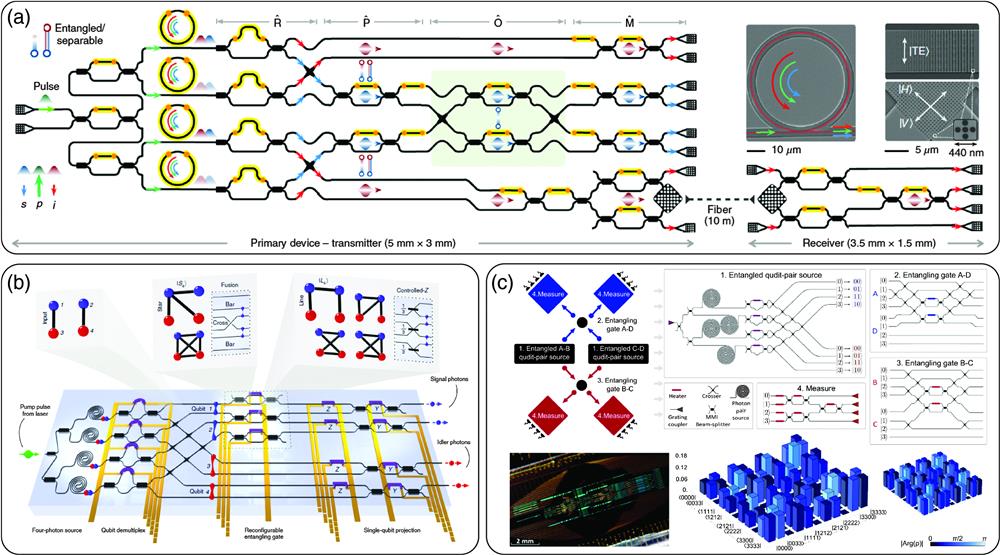
Fig. 2. On-chip generation of multiphoton entanglement. (a) Generation of four-photon four-qubit genuine GHZ entangled states on a silicon photonic chip. Two photon-pairs with high purity and indistinguishability were produced by an array of microring resonators. Then, a reconfigurable fusion entangling operator was performed on two indistinguishable photons, generating the genuine four-photon GHZ entangled state. Entanglement witness confirmed the genuine multiphoton entanglement. (b) Generation of four-photon four-qubit cluster states on a silicon photonic chip. An entangling gate could be tuned to perform either a fusion operator or controlled- operator, by which the linear- and star-cluster states were created. Thanks to the highly reconfigurable photonics chip, all types of four-photon graph states were prepared. Nonlocality of the multiphoton state was verified by the Mermin test. (c) Generation of four-photon eight-qubit graph states on a silicon photonic chip, in which each of the four-dimensional qudits was remapped into two qubits. High-dimensional entangling gates were ultilized to generate four-photon four-dimensional entanglement from two pairs of two-photon four-dimensional entangled states. Error-corrected qubits were used to implement quantum computational algorithms. When running the phase-estimation algorithm with error protection, the success rate would raise from 62.5% to 95.8%. Panels reproduced from: (a) Ref. 62, Springer Nature; (b) Ref. 77, Springer Nature; and (c) Ref. 82, Springer Nature.
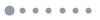
Fig. 3. On-chip generation of multidimensional entanglement. (a) Generation of frequency-bin encoded multidimensional entangled state. An integrated microring resonator was used to produce a pair of frequency-entangled photons with up to 10-dimensions. High-dimensional gate operations in the frequency domain were executed on qudits, demonstrating qudit two-photon interference and qudit tomography. (b) Generation of path-encoded multidimensional entanglement on a large-scale programmable silicon-photonic chip, where 16 identical SFWM single-photon sources and qudit operation/analyzing networks were all integrated monolithically. Each single-photon source would generate a pair of highly indistinguishable photons. By this means, this chip can create, control, and measure entangled states. Genuine multidimensional entanglement was verified by quantum state tomography, and experimental violation of generalized Bell inequality and steering inequality. (c) Multipath wave-particle duality experiment on a large-scale silicon chip. The state-process entanglement technique was adopted to implement the quantum-controlled generalized Hadamard operations. The process (wave/particle) of the target photon going through would be coherently controlled by the state of the control photon. The generalized multipath wave-particle duality was demonstrated qualitatively and quantitatively. Panels reproduced from: (a) Ref. 51, Springer Nature; (b) Ref. 43, AAAS; and (c) Ref. 90, Springer Nature.
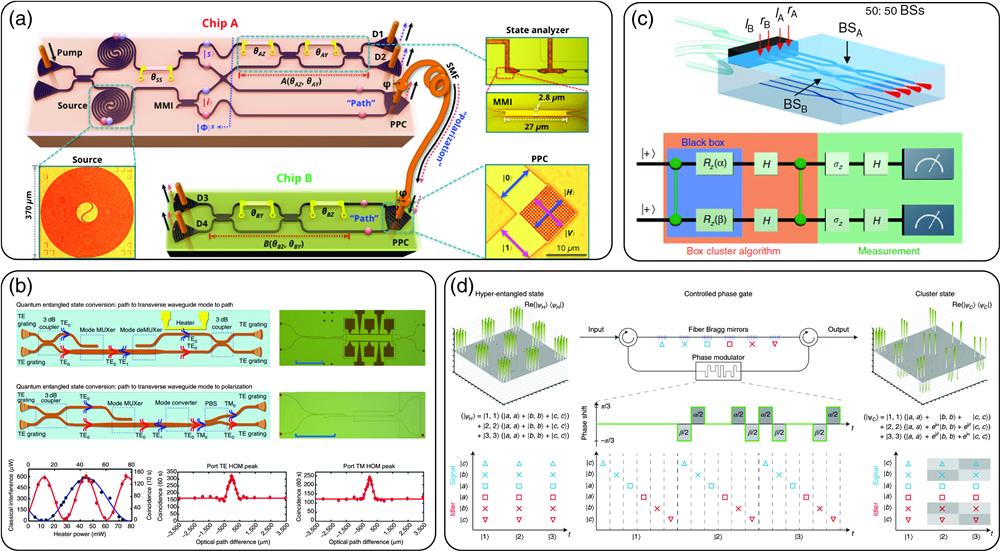
Set citation alerts for the article
Please enter your email address