Kun Huang, Yinqi Wang, Jianan Fang, Huaixi Chen, Minghang Xu, Qiang Hao, Ming Yan, Heping Zeng, "Highly efficient difference-frequency generation for mid-infrared pulses by passively synchronous seeding," High Power Laser Sci. Eng. 9, 010000e4 (2021)

Search by keywords or author
- High Power Laser Science and Engineering
- Vol. 9, Issue 1, 010000e4 (2021)
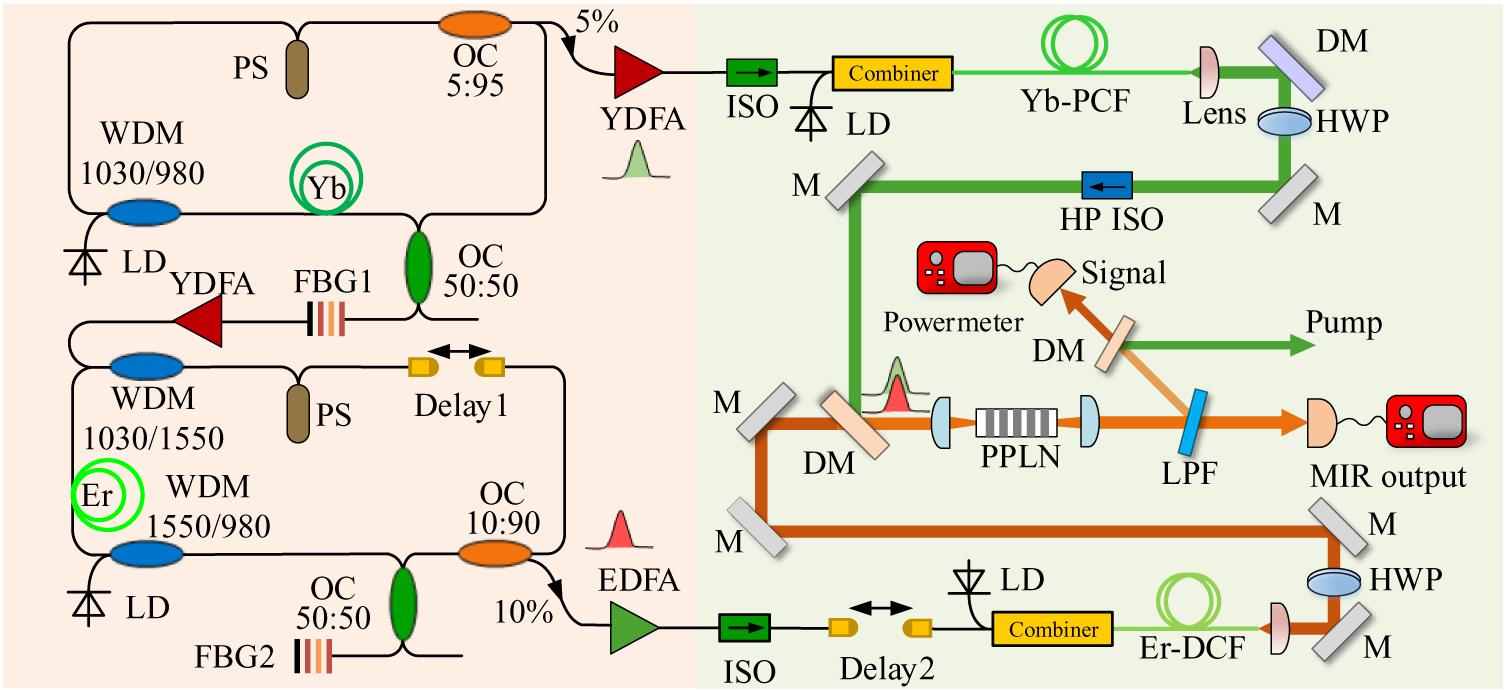
Fig. 1. Experimental schematic for mid-infrared generation based on passively synchronized ultrafast fiber laser system. The pump and signal pulses originated from mode-locked Yb- and Er-doped fiber lasers, respectively. After cascaded fiber amplifiers, the two-color pulses were steered into a PPLN crystal for implementing difference-frequency generation. Consequently, the average power and conversion efficiency for the MIR output could be effectively improved due to the synchronous seeding. LD: laser diode; WDM: wavelength division multiplexer; Yb/Er: ytterbium/erbium-doped gain fiber; OC: optical coupler; PS: phase shifter; FBG: fiber Bragg grating; PCF: photonic crystal fiber; DCF: double-clad fiber; DM: dichroic mirror; HWP: half-wave plate; M: mirror; HP ISO: high-power isolator; LPF: long-pass filter; PPLN: periodically-poled lithium niobate crystal.
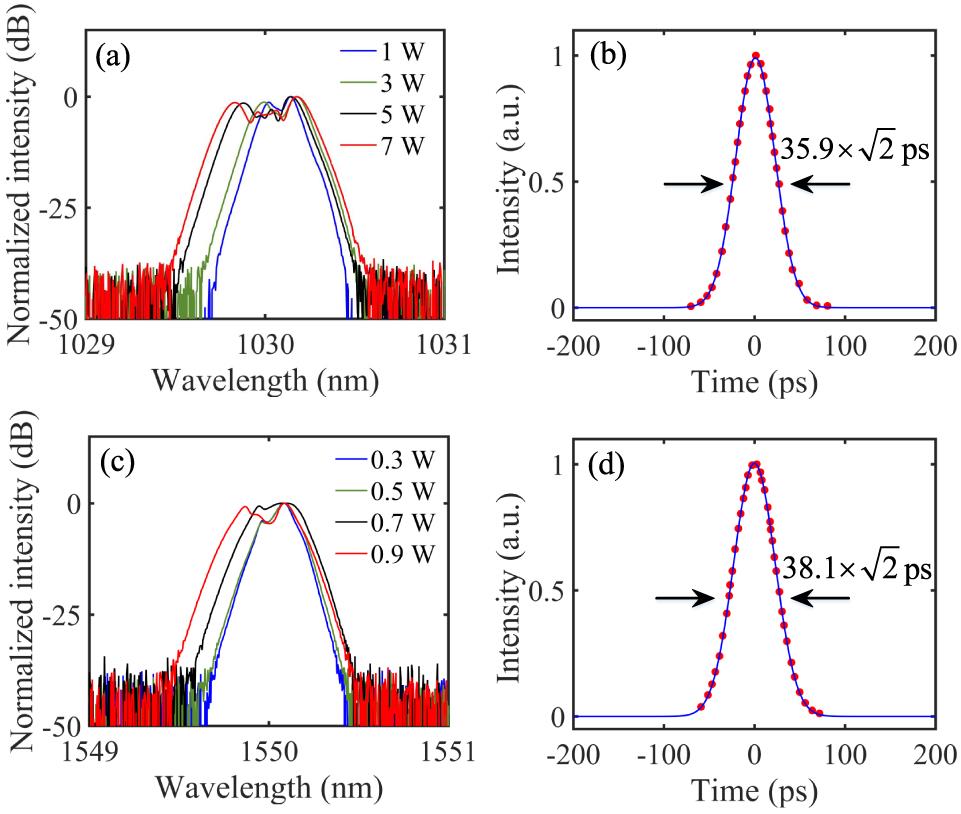
Fig. 2. Experimental characterization of output pulses from (a), (b) pump and (c), (d) signal after two-stage fiber amplifiers, including (a), (c) the measured optical spectra and (b), (d) corresponding auto-correlation traces. Note that the traces given in (b) and (d) were measured at the average power of 7 and 0.9 W, respectively. The actual intensity profiles are scaled down by a factor of
under an assumption of Gaussian pulses.

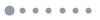
Fig. 3. (a) MIR spectra under different pump power. The signal power was kept at 900 mW. (b) Power stability of the mid-infrared output.
indicates the relative fluctuation. (c) MIR beam image at the near field as well as two section profiles along orthogonal axes. (d) Evolution of MIR beam waists along the propagation distance. Note that the central position was defined at the focal point.

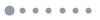
Fig. 4. Generated mid-infrared power and corresponding conversion efficiency vary as functions of the pump power. Note that the conversion efficiency was defined as total power of down-converted fields divided by the initial pump power. Connecting lines are only used to guide the eye.
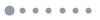
Fig. 5. (a) Signal gain against input signal power for different pump power. (b) Generated MIR power versus injected signal power under various settings of pump power. Solid lines are used to guide the eyes only.
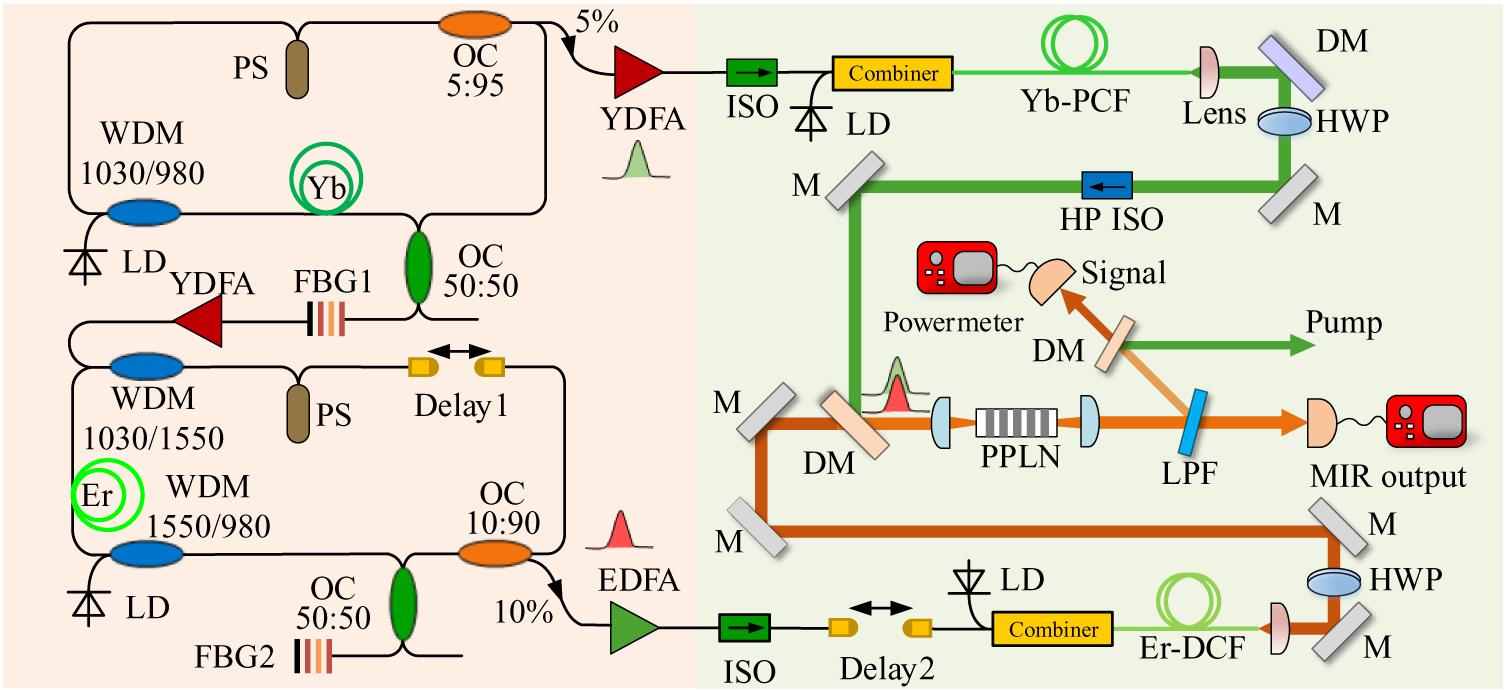
Set citation alerts for the article
Please enter your email address