Wagner Tavares Buono, Andrew Forbes. Nonlinear optics with structured light[J]. Opto-Electronic Advances, 2022, 5(6): 210174

Search by keywords or author
- Opto-Electronic Advances
- Vol. 5, Issue 6, 210174 (2022)
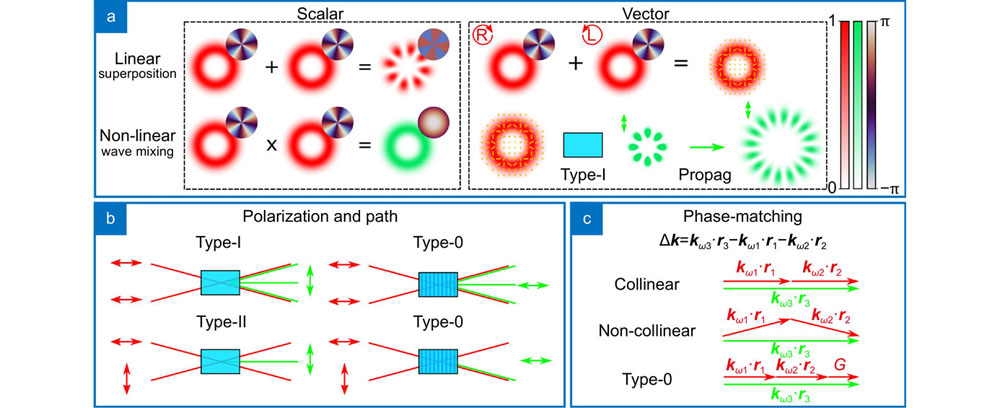
Fig. 1. Linear and nonlinear processes. Using second harmonic generation (SHG), we illustrate the differences between linear and nonlinear processes. (a ) Linear processes produce an output mode that is the addition of two input spatial modes of light, while SHG produces the product of the two modes. The linear superposition of two different modes with orthogonal polarization states generates a vector beam, which has a inhomogeneous polarization state. The polarization profile is represented as yellow lines across the transverse profile. In SHG, and wave mixing in general, the polarization profile will dictate where wave mixing happens and thus alter directly the spatial profile. In (b ) we show exemplify how path can also be controlled via polarization and the different phase matching conditions of crystals, including the periodic poling of type-0. The mechanism which allows these interactions is sketched in (c ). Phase-matching is the condition necessary for wave mixing to occur and exploits birefringence (types I and II) or periodical polling (type-0) to achieve it.
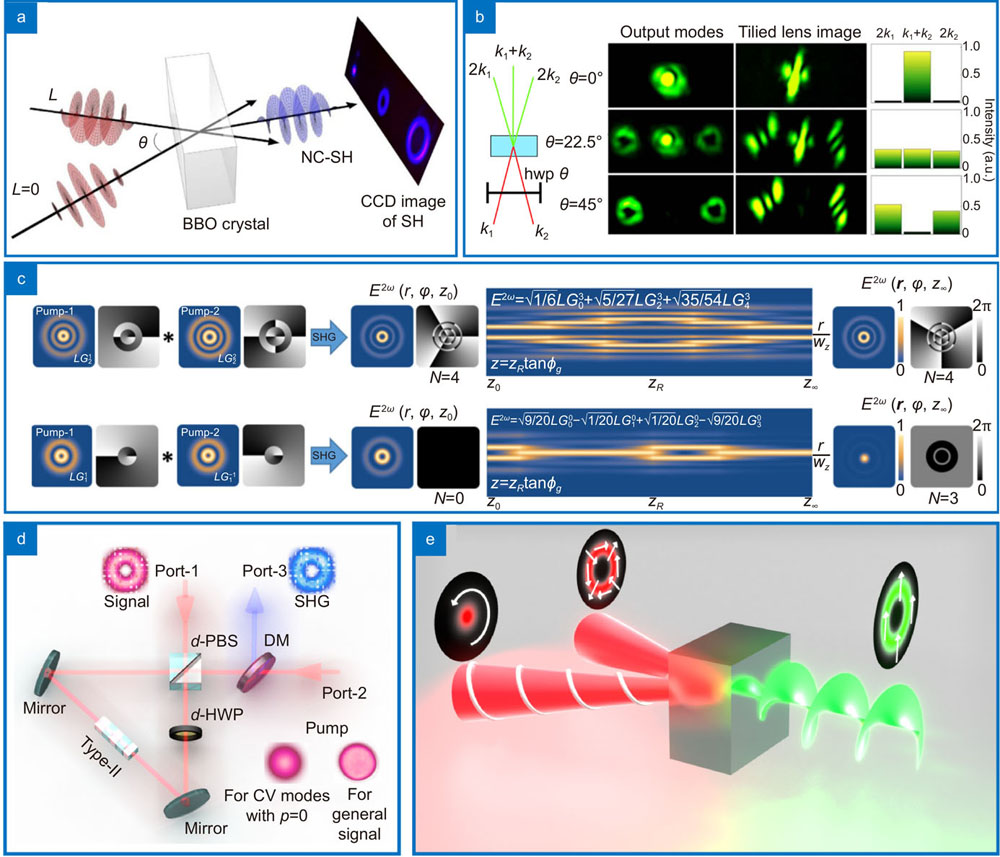
Fig. 2. Wave mixing with different degrees of freedom. In (a ), the authors show OAM algebra in noncollinear SHG. When type-II phase-matching is used, the same noncollinear geometry allows for polarization switching, shown in (b ). This effectively couples multiple degrees of freedom in a single process: path, polarization, radial and angular transverse structures. The radial selection rules of LG modes in wave mixing are demonstrated in (c ). There is a intrinsic relation between the radial and angular degrees of freedom, which is manifested in the propagation dependence of the spatial profiles. In (d ), a experimental scheme using a Sagnac interferometer achieves faithful frequency conversion of vector light. Spin and orbital angular momentum are combined in second harmonic generation in (e ). Figure repoduced with permission from: (a) ref.48, Springer Nature; (b) ref.50, © Optica Publishing Group; (c) ref.54, © American Physical Society; (d) ref.69, American Physical Society; (e) ref.70, under aCreative Commons Attribution 4.0 International License
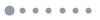
Fig. 3. Nonlinear Holography. In (a ) the structuring of the medium is illustrated: the fundamental field is always the same, but the medium is not. The selective inversion of the electric domain across the transverse plane creates different spatial structures in the second harmonic field. The periodical transverse structure is responsible for multiple phase matching mechanisms, both longitudinally and transversely. In (b ) it is shown how non-collinear SHG can transfer a specific intensity pattern from one wavelength to the other. First row shows the imaging arrangement and the second column shows the phase-matching conditions and an example of output modes. Right below is a experimental demonstration that this can be used for real-time frequency conversion of computer generated holograms. Figure repoduced from: (a) ref.130, © American Physical Society; (b) ref.158, © Optica Publishing Group.
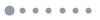
Fig. 4. Nonlinear optics enabled metasurfaces. These devices were shown to enable non-trivial interactions while frequency converting beams. In (a ) a SHG process coupling SAM and OAM. The combination of frequency conversion with holography creates metasurfaces with metalensing properties in (b )144. An application taking advantage of the high damage threshold of these materials can be seen in (c )111 where the inclusion of a metasurface inside an optical cavity creates a laser with OAM from the source. Figure repoduced with permission from: (a) ref.143, © American Chemical Society; (b) ref.144, under a Creative Commons Non-Commercial No Derivative Works (CC-BY-NC-ND) Attribution License; (c) ref.111, Springer Nature.
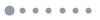
Fig. 5. Higher order process. In the generation of high harmonic orders, it is possible to generate beams of many different OAM from just two different inputs, as depicted in (a ). The process of writting and reading optical memory is depicted in (b ) and the diference in time scales depending on the order of the nonlinear process in (c ). In (d ) it is demonstrated robust self-trapping of a bright vortex beam by exploiting higher order nonlinearities of odd orders. Figure repoduced from: (a) ref.209, Springer Nature; (b) ref.191, © Optica Publishing Group; (c) ref.192, © Optica Publishing Group; (d) ref.204, © American Physical Society.
|
Table 1. Behaviour of various structures of light in second order nonlinear wave mixing. Here, nx/ny are the indices for HG modes, ℓ, p are the azimuthal/radial indices for LG modes and p/m are the parameters for Ince-Gaussian modes. Indices with primes, such as ℓ′′ are of fundamental field modes and the ones without are of the frequency generated.
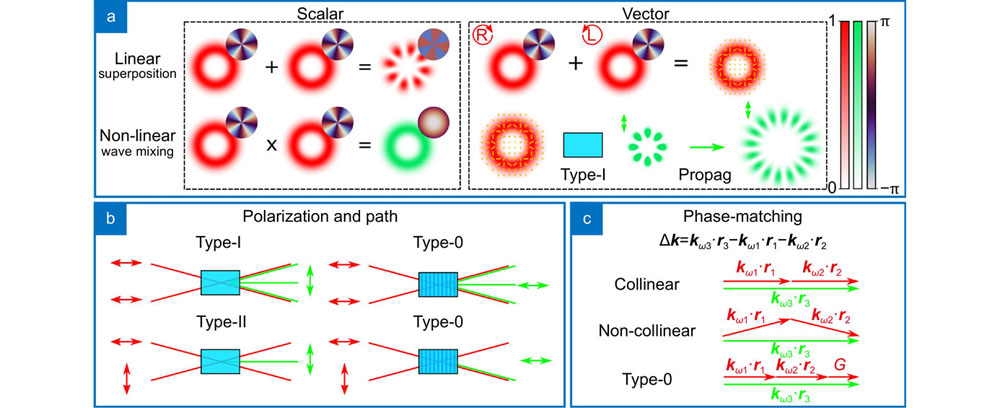
Set citation alerts for the article
Please enter your email address