Author Affiliations
1Institute of Brain-Inspired Intelligence, National Laboratory of Solid State Microstructures, School of Physics, Collaborative Innovation Center of Advanced Microstructures, Nanjing University, Nanjing 210008, China2Institute of Interdisciplinary Physical Sciences, School of Science, Nanjing University of Science and Technology, Nanjing 210094, Chinashow less
Moiré heterostructures, where the constituent 2D materials are stacked vertically at a small angle, feature long-wavelength interference pattern at the van der Waals interfaces. One typical example is twisted bilayer graphene. In this moiré heterostructure, the emergence of moiré superlattices can effectively reconstruct the energy bands into dispersionless flat bands at a special twisted angle (so-called ‘magic angle’), by folding the original bands into the mini-Bouillon zone and enhancing interlayer quantum coherence. Such flattened bands can strongly suppress electronic kinetic energy and thus the electron correlation dominates over the kinetic energy, leading to correlated insulating states[1,2] and unconventional superconductivity[3,4]. Not limited to bilayer graphene, flat bands and strongly correlated quantum states have also been observed in moiré heterostructures based on multi-layer graphene[5-12] and semiconducting transition metal dichalcogenides (TMDs)[13-23], manifesting themselves as versatile solid-state platforms for simulating Hubbard models and exploring novel quantum criticalities[18,20,24]. Meanwhile, the nontrivial topology of the flat bands in twisted graphene moiré heterostructures is characterized by nonzero valley Chern numbers and gives rise to opposite orbital magnetic moments at the two valleys. The valley degeneracy can be further lifted to exhibit ferromagnetic Chern insulators by applying magnetic fields[25,26], electric fields[8,10], or by using the h-BN substrate to create a sublattice unbalanced potential[27,28]. By stacking two-dimensional materials to form rhombohedral crystal lattices, periodic ferroelectric domains with opposite out-of-plane polarization, which is coined as “sliding ferroelectricity”, can be observed in parallelly stacked twisted h-BN[29,30] and hexagonal TMDs[31,32]. Notably, the ferroelectricity reported in these moiré systems is different from that observed in bilayer graphene/h-BN moiré superlattices, where the origin is claimed to arise from the electron correlation[33-35]. In addition to the aforementioned emergent quantum states, there is much intensive research interest in the highly localized moiré excitons arising from strong moiré potential that reconstructs the exciton dispersion in TMD moiré heterostructures. The advent of moiré heterostructures as new artificial quantum materials not only shifts the landscape of condensed matter physics but also serves as a promising platform for future technology (Fig. 1).
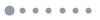
Figure 1.Quantum states, tuning knobs and possible device applications of moiré heterostructures.
The superiority of moiré heterostructures for exploring fundamental physics and future technology lies in their capability of fine control over the emerging quantum states through multiple tuning knobs. The electrostatic gating is the most important tuning knob. On the one hand, it allows for in-situ varying the charge density in the system, hence the filling factor of moiré flat bands, which enables clean and continuous doping compared with the chemical doping commonly used in conventional strongly correlated systems. On the other hand, an asymmetrical gating on the heterostructure generates a perpendicular displacement field, and significantly alters the energy band structures as well as the interaction strength, thus providing a unique tuning knob for directly tuning the electron correlation[5-12,21,36,37], which is inaccessible in conventional strongly correlated materials. The ferroelectricity in moiré heterostructures can be switched by using electrostatic gating, which is crucial for realizing ultrahigh-density memory and ultrahigh-accuracy in-memory computing. As another type of spontaneous polarization, the orbital magnetic polarization can also be reversed by the electric gating in twisted monolayer-bilayer graphene due to the spontaneous switching of valley polarization upon doping[8]. Notably, the orbital magnetism reversal can also be achieved in twisted bilayer graphene using a pulsed bias current to shift the energy of edge states of opposite chirality[27,28]. It is worth noting that current-controlled switching can cooperate with gate-controlled switching, which facilitates the development of multi-mode electrical control of magnetism. Meanwhile, a steady bias current has been adopted to access unconventional out-of-equilibrium criticalities[38], estimate the Dirac Fermi velocity[39] and characterize the electronic nematicity of correlated states[40] in graphene moiré heterostructures. Besides, the anisotropic correlated phases can also be probed by an in-plane magnetic field and in-plane strain. The in-plane magnetic field can polarize the spins in graphene-based moiré heterostructures owing to the negligible spin-orbit coupling (SOC). This facilitates the determination of the symmetry of superconducting pairing[40] and the continuous evolution of internal degrees of freedom from SU(4) symmetry to SU(2) symmetry, providing a unique setting for studying the intricate interplay between intrinsic degrees of freedom and electron correlation. The rotational symmetry of the underlying moiré superlattice may be directly altered by the in-plane strain, providing insights into the symmetry of superconducting pairing and correlated insulators without affecting the spins. Moreover, the in-plane strain can generate Berry curvature dipole in the topological flat bands, which can be characterized by the nonlinear Hall effect.
With the exotic quantum states, excellent tunability and diverse experimental probe techniques, moiré heterostructures hold great potential for becoming solid-state quantum simulators[41]. A quantum simulator was first proposed by Feynman in the early 80s as a paradigm to understand the ground states and evolution laws of quantum many-body systems by building controllable quantum systems that follow the same physical rules. Remarkably, quantum simulators have shown their capability in investigating electron systems with strong correlation, which has been a huge challenge even utilizing the most powerful computers. The current works of moiré quantum simulators mainly focus on twisted TMDs, in which the simulation of single-band Hubbard model physics with SU(2) internal symmetry[15] has been identified by both optical spectroscopy and transport measurements near half-filling of the flat band. Recently, the Hubbard model simulator has been extended to SU(4) symmetry by fabricating chiral-stacked twisted double bilayer graphene (TDBG), observing the generalized isospin Wigner state. The perpendicular electrical field-controlled electron interaction and the small contact resistance in this carbon-based quantum simulator enable the in-situ investigation of quantum melting of the generalized Wigner crystal, demonstrating the existence of a critical intermediate phase. Especially, the extremely weak SOC facilitates the continuous evolution of the internal degrees of freedom of this quantum simulator from SU(4) to SU(2) by adopting the in-plane magnetic field[24], which offers an unprecedented opportunity for studying the interplays of electron correlation and the internal degrees of freedom in the vicinity of quantum phase transitions. There are still several challenges in broadening the scope of the research of moiré quantum simulators. First, the current moiré quantum simulators mainly simulate the single-band Hubbard model, and thus building quantum simulators for other models is urgently required. The models of particular interest include bilayer Hubbard model[42], Bose-Hubbard model, multiband Hubbard model, anisotropic Hubbard model, and those beyond Hubbard models, such as the Kondo model for heavy fermions[43], exotic quantum magnetic models for quantum spin liquid[44,45], and twisted superconducting models for the crossover between strong coupling and weak coupling of superconductivity[41]. Second, although a plethora of tuning knobs is readily available, the control tools used in the studies of moiré quantum simulators are only limited to electrical gating and magnetic fields, while the bias current, strains and dielectric environment, etc., are still not exploited. The strain modification of the superlattices and bias current might become viable routes to realizing anisotropic modulation of interaction and out-of-equilibrium quantum phenomena. Another urgently required tuning knob is to have fine control over the electron on each site and the inter-site coupling, which is of great importance for simulating impurity-related effects like local Kondo screening and Anderson localization. Finally, the read-out of quantum states in the moiré quantum simulators is mainly based on electrical transport and optical measurements, which lacks the capability of directly characterizing the electronic band structures and local information of wavefunctions and spin texture. While high-resolution angle-resolved photoemission spectroscopy for sub-micron-sized samples (micron-ARPES) is promising to probe the band structure[46], the strategy of local read-out of quantum states[19] and their evolution are still not known. The local read-out as well as the local control of the individual sites are the key steps for the further application of moiré heterostructures in general quantum simulation[47], and thus deserve more attention in future research.
In addition to the huge potential in quantum simulations, the moiré heterostructures are also promising for future computing beyond conventional CMOS technologies, which would revolutionize the traditional way that the information is sensed, memorized and processed as well as transmitted. The unique quantum states in the quantum materials can be used for encoding the sensed information and the tuning of the state variables by external stimuli, like electrical fields, light or strain, allows for the implementation of the computing, such as in-sensor computing, in-memory computing, neuromorphic computing and quantum computing. Recently, intelligent sensing of infrared light has been demonstrated by employing bulk photovoltaic effect in TDBG devices[48], where the nonlinear photovoltage depends on the gate-controlled quantum geometry as well as the incident infrared light. With the in-sensor computing paradigm, full Stokes parameters of incident light can be decoded from the measured photovoltages in the TDBG devices. Note that the external stimulus with multiple parameters can be inferred by exploiting the diverse tunability of flat band topology and the appropriate machine-learning algorithm, which can be trained by using data collected from a single moiré heterostructure optoelectronic device. Such an alliance between moiré heterostructures and in-sensor computing enables inferences of analog information, for example, external strain and magnetic fields, without using bulky equipment. Specifically, the nonlinear Hall effect present in the moiré heterostructures might facilitate the parameterization of in-plane strain, in terms of magnitude and orientation, since the transverse nonlinear voltages depend on the gate-controlled Berry curvature dipole and the rotational symmetry-breaking strain. The emergent ferroelectricity and orbital magnetism in moiré heterostructures provide platforms for exploring ultrahigh-accuracy in-memory computing. The ferroelectric twisted TMDs and ferroelectric graphene superlattices are attributed to interlayer charge transfer and thus the charge polarization is dependent on both displacement field and charge density. This unique mechanism could be exploited for developing non-volatile memory with multilevel resistance states. Meanwhile, the gate-controlled or current-induced reversal of magnetic polarization in moiré orbital magnets is promising for nearly unlimited endurance and ultra-low power computing. Beyond the in-memory computing, superconducting diodes recently reported in the moiré heterostructure serve as a new platform for realizing ultrafast neuromorphic computing. Traditionally, the spikes of the neuron are emulated by the current-induced transition between the superconducting state and the normal state in specially designed superconducting circuits. Instead, in a superconducting diode device, the nonreciprocal transport behavior can be tailored by current-induced magnetization switching, resulting in tunable generation of spikes with nonvolatile memory, which could potentially be used for neuromorphic computing. Last but not least, the moiré superlattice potential endows the interlayer excitons a unique spin-dependent complex hopping, leading to giant spin-orbit splitting in the exciton bands. Such long-life-time moiré excitons can be tuned by out-of-plane electric fields and in-plane strain. In this way, a qubit may be encoded with the spin state of moiré excitons for quantum computing[49].
Despite the huge potential in future computing, there are some key challenges associated with device physics, integration technology as well as circuit models of the moiré heterostructures. First, the studies of moiré heterostructure mainly focus on the new quantum states and their tunability, while there are only a few works in this field. It is challenging to utilize the exotic physical phenomena emergent in the moiré heterostructures to design new quantum devices desirable for future computing, since the working mechanisms of the devices are dependent on both materials and the device structures. Second, the quantum devices based on the moiré heterostructures require new integration technology to retain their practical advantages, since the small energy scale of flat bands restricts most strong-correlation effects to low temperatures. Third, it is important to develop reliable models to evaluate the real advantages of integrated systems of moiré heterostructures in future computing.
References
[1] Y Cao, V Fatemi, A Demir et al. Correlated insulator behaviour at half-filling in magic-angle graphene superlattices. Nature, 556, 80(2018).
[2] X Lu, P Stepanov, W Yang et al. Superconductors, orbital magnets and correlated states in magic-angle bilayer graphene. Nature, 574, 653(2019).
[3] Y Cao, V Fatemi, S Fang et al. Unconventional superconductivity in magic-angle graphene superlattices. Nature, 556, 43(2018).
[4] M Oh, K P Nuckolls, D Wong et al. Evidence for unconventional superconductivity in twisted bilayer graphene. Nature, 600, 240(2021).
[5] Y Cao, D Rodan-Legrain, O Rubies-Bigorda et al. Tunable correlated states and spin-polarized phases in twisted bilayer–bilayer graphene. Nature, 583, 215(2020).
[6] G Chen, A L Sharpe, E J Fox et al. Tunable correlated Chern insulator and ferromagnetism in a moiré superlattice. Nature, 579, 56(2020).
[7] X Liu, Z Hao, E Khalaf et al. Tunable spin-polarized correlated states in twisted double bilayer graphene. Nature, 583, 221(2020).
[8] H Polshyn, J Zhu, M A Kumar et al. Electrical switching of magnetic order in an orbital Chern insulator. Nature, 588, 66(2020).
[9] G Chen, L Jiang, S Wu et al. Evidence of a gate-tunable Mott insulator in a trilayer graphene moiré superlattice. Nature Phys, 15, 237(2019).
[10] S Chen, M He, Y H Zhang et al. Electrically tunable correlated and topological states in twisted monolayer–bilayer graphene. Nature Phys, 17, 374(2021).
[11] C Shen, Y Chu, Q Wu et al. Correlated states in twisted double bilayer graphene. Nature Phys, 16, 520(2020).
[12] S Xu, M M Al Ezzi, N Balakrishnan et al. Tunable van Hove singularities and correlated states in twisted monolayer–bilayer graphene. Nature Phys, 17, 619(2021).
[13] E C Regan, D Wang, C Jin et al. Mott and generalized Wigner crystal states in WSe2/WS2 moiré superlattices. Nature, 579, 359(2020).
[14] Y Shimazaki, I Schwartz, K Watanabe et al. Strongly correlated electrons and hybrid excitons in a moiré heterostructure. Nature, 580, 472(2020).
[15] Y Tang, L Li, T Li et al. Simulation of Hubbard model physics in WSe2/WS2 moiré superlattices. Nature, 579, 353(2020).
[16] L Wang, E M Shih, A Ghiotto et al. Correlated electronic phases in twisted bilayer transition metal dichalcogenides. Nature Mater, 19, 861(2020).
[17] Y Xu, S Liu, D A Rhodes et al. Correlated insulating states at fractional fillings of moiré superlattices. Nature, 587, 214(2020).
[18] A Ghiotto, E M Shih, G S S G Pereira et al. Quantum criticality in twisted transition metal dichalcogenides. Nature, 597, 345(2021).
[19] H Li, S Li, E C Regan et al. Imaging two-dimensional generalized Wigner crystals. Nature, 597, 650(2021).
[20] T Li, S Jiang, L Li et al. Continuous Mott transition in semiconductor moiré superlattices. Nature, 597, 350(2021).
[21] T Li, S Jiang, B Shen et al. Quantum anomalous Hall effect from intertwined moiré bands. Nature, 600, 641(2021).
[22] J Gu, L Ma, S Liu et al. Dipolar excitonic insulator in a moiré lattice. Nature Phys, 18, 395(2022).
[23] Z Zhang, E C Regan, D Wang et al. Correlated interlayer exciton insulator in heterostructures of monolayer WSe2 and moiré WS2/WSe2. Nature Phys, 18, 1214(2022).
[24] Q Li, B Cheng, M Chen et al. Tunable quantum criticalities in an isospin extended Hubbard model simulator. Nature, 609, 479(2022).
[25] S Wu, Z Zhang, K Watanabe et al. Chern insulators, van Hove singularities and topological flat bands in magic-angle twisted bilayer graphene. Nature Mater, 20, 488(2021).
[26] Y Saito, J Ge, L Rademaker et al. Hofstadter subband ferromagnetism and symmetry-broken Chern insulators in twisted bilayer graphene. Nature Phys, 17, 478(2021).
[27] M Serlin, CL Tschirhart, H Polshyn et al. Intrinsic quantized anomalous Hall effect in a moiré heterostructure. Science, 367, 900(2020).
[28] A L Sharpe, E J Fox, AW Barnard et al. Emergent ferromagnetism near three-quarters filling in twisted bilayer graphene. Science, 365, 605(2019).
[29] M Vizner Stern, Y Waschitz, W Cao et al. Interfacial ferroelectricity by van der Waals sliding. Science, 372, 1462(2021).
[30] K Yasuda, X Wang, K Watanabe et al. Stacking-engineered ferroelectricity in bilayer boron nitride. Science, 372, 1458(2021).
[31] X Wang, K Yasuda, Y Zhang et al. Interfacial ferroelectricity in rhombohedral-stacked bilayer transition metal dichalcogenides. Nature Nanotechnol, 17, 367(2022).
[32] A Weston, E G Castanon, V Enaldiev et al. Interfacial ferroelectricity in marginally twisted 2D semiconductors. Nature Nanotechnol, 17, 390(2022).
[33] D R Klein, L Q Xia, D MacNeill et al. Electrical switching of a moiré ferroelectric superconductor. arXiv: 2205.04458(2022).
[34] R Niu, Z Li, X Han et al. Giant ferroelectric polarization in a bilayer graphene heterostructure. Nature Commun, 13, 6241(2022).
[35] Z Zheng, Q Ma, Z Bi et al. Unconventional ferroelectricity in moiré heterostructures. Nature, 588, 71(2020).
[36] J M Park, Y Cao, K Watanabe et al. Tunable strongly coupled superconductivity in magic-angle twisted trilayer graphene. Nature, 590, 249(2021).
[37] Z Hao, A M Zimmerman, P Ledwith et al. Electric field–tunable superconductivity in alternating-twist magic-angle trilayer graphene. Science, 371, 1133(2021).
[38] A I Berdyugin, N Xin, H Gao et al. Out-of-equilibrium criticalities in graphene superlattices. Science, 375, 430(2022).
[39] H Tian, S Che, T Xu et al. Evidence for flat band dirac superconductor originating from quantum geometry. arXiv: 2112.13401(2021).
[40] Y Cao, D Rodan-Legrain, J M Park et al. Nematicity and competing orders in superconducting magic-angle graphene. Science, 372, 264(2021).
[41] D M Kennes, M Claassen, L Xian et al. Moiré heterostructures as a condensed-matter quantum simulator. Nature Phys, 17, 155(2021).
[42] Y Xu, K Kang, K Watanabe et al. A tunable bilayer Hubbard model in twisted WSe2. Nature Nanotechnol, 17, 934(2022).
[43] W Zhao, B Shen, Z Tao et al. Gate-tunable heavy fermions in a moiré Kondo lattice. arXiv: 2211.00263(2022).
[44] C Wang, Y Gao, H Lv et al. Stacking domain wall magnons in twisted van der Waals magnets. Phys Rev Lett, 125, 247201(2020).
[45] K Hejazi, Z X Luo, L Balents. Noncollinear phases in moiré magnets. Proceedings of the National Academy of Sciences, 117, 10721(2020).
[46] Y Li, S Zhang, F Chen et al. Observation of coexisting dirac bands and moiré flat bands in magic‐angle twisted trilayer graphene. Adv Mater, 34, 2205996(2022).
[47] S B Bravyi, A Y Kitaev. Fermionic quantum computation. Annals of Physics, 298, 210(2002).
[48] C Ma, S Yuan, P Cheung et al. Intelligent infrared sensing enabled by tunable moiré quantum geometry. Nature, 604, 266(2022).
[49] K F Mak, D Xiao, J Shan. Light–valley interactions in 2D semiconductors. Nature Photonics, 12, 451(2018).