
- Infrared and Laser Engineering
- Vol. 50, Issue 5, 20200287 (2021)
Abstract
0 Introduction
Quantum dots belong to quasi-zero-dimensional structure, and their dimensions are all within the range of 1-20 nm. Due to the quantum surface effect, the proportion of atoms on the surface is large, leading to the absence of other atoms in the periphery of some surface atoms[
Typical materials, CdTe quantum dots synthesized by the hydrothermal method have special nonlinear optical and ultrafast dynamics characteristics such as narrow laser emission spectrum, continuous distribution of excitation wavelength, and high photochemical stability[
In order to further research the preparation and fluorescence characteristics, in this work, the 10 kinds of CdTe/CdS and CdTe/ZnS core-shell semiconductor quantum dots with different core sizes and shell thicknesses were synthesized by the TGA passivation surface method. The influences of PH value on reaction speed and time on the shell thickness during the preparation of CdTe/CdS and CdTe/ZnS quantum dots were compared and analyzed. The effects of the shell thickness and the core size on the absorption spectrum and the fluorescence properties were investigated, which provided a theoretical basis for the preparation of core-shell structure and the study of photophysical properties.
1 Experiment
1.1 Preparation of the NaHTe
A three-necked, round-bottomed flask was used as the reaction vessel. A glass tube at the first flask-mouth to inject high-purity N2 into the reaction liquid, and the pressure in the three-necked flask was increased at the beginning of the reaction to prevent air entering. The gas was exhausted during the second flask-mouth. The glass tube was connected to the rubber tube and inserted into another beaker filled with silicone oil, to prevent the air from being sucked into the reaction flask while exhausting. It was equipped with a rubber plug and a glass tube for adding drugs at the last flask-mouth. The rubber plug was used to seal the glass tube after adding, and the NaHTe solution was extracted after completion of the reaction in the syringe. The specific operation was described as follows: first, connected the device and injected N2; second, added 30 ml deionized water and injected N2 into the water after 5 minutes; third, magnetic stirring for 10 minutes, the three-necked flask was in an oxygen-free environment; then added 2.5 g Te powder and 1.8 g NaBH4 through the stopper of the glass tube, and sealed the glass tube with a rubber plug quickly. To prevent air entering, a syringe was used and a long needle was inserted into a rubber plug at the third opening of the flask in the process of extracting the NaHTe solution after the reaction.
Using the device, the reaction at room temperature was achieved. The exhaust system was sufficient to handle hydrogen generated at the high speed, as well as the N2 provided cooling. In this paper, the new preparation method has successfully reduced the traditional harsh conditions of reaction from 10 hours at 5 ℃ to 1 hour at the room temperature and improved the quality of the NaHTe solution. The preparation quality and speed were increased, and Te source was the furthest avoided from being polluted by oxidation to the greatest extent.
1.2 Synthesis of the CdTe cores
The synthetic procedure was instructed according to the method mentioned in Ref.[12] with minor modification Typically, took 500 mL of deionized water and put it into a 1000 mL three-necked flask, then added0.4565 g of CdCl2•2.5 H2O, 0.310 ml of TGA, passed in N2, and stirred. Adjusted the pH of the solution to 9 with 1 mol/L NaOH. Took 0.612 ml of the NaHTe solution which was prepared and added it into a three-necked flask, adjusted the heating temperature to 100 ℃, condensed and refluxed for 2 hours to complete the preparation of the CdTe core.
Repeated the above process twice and adjusted the pH value to 10 and 11 respectively. The condensation reflux was adjusted accordingly for 6 hours and 10 hours to prepare the CdTe core. The sample was observed under the 365 nm ultraviolet light and the color was shown in Tab.1.
Sample number | Quantum dots | Size/nm | Core color | Cladding time |
S1 | CdTe/CdS-1 | 3.2 | Orange | 30 min |
S2 | CdTe/CdS-2 | 3.3 | Orange | 1 h |
S3 | CdTe/CdS-3 | 3.4 | Orange | 2 h |
S4 | CdTe/CdS-4 | 3.0 | Yellow | 30 min |
S5 | CdTe/CdS-5 | 3.1 | Yellow | 1 h |
S6 | CdTe/CdS-6 | 2.6 | Green | 30 min |
S7 | CdTe/CdS-7 | 2.7 | Green | 1 h |
S8 | CdTe/CdS-8 | 2.8 | Green | 2 h |
S9 | CdTe/ZnS-9 | 3.5 | Orange | 30 min |
S10 | CdTe/ZnS-10 | 3.6 | Orange | 1 h |
Table 1. Ten kinds of the core-shell structure quantum dots
1.3 Preparation of the CdTe/CdS core-shell quantum dots
Took 100 mL CdTe solution and put it into 250 mL three-necked flask, passed in the N2 and stirred. Weighed 0.0122 g thiourea in a three-necked flask and recovered the pH of the solution to 10 with 1 mol/L NaOH. Set the temperature for 90 ℃ and started the timer when the temperature reached 90 ℃. The reflux time was 30 min, 1 h and 2 h, respectively. After the reaction, the three-necked flask was cooled to room temperature quickly with cold water.
The obtained the CdTe/CdS quantum dots solution was put into the centrifuge tube, and the ratio of the CdTe/CdS quantum dots to the ethanol in the solution was 1∶2 to 1∶4 (volume ratio), and centrifuged in a high-speed centrifuge, with the rotation speed of 10000 r/min and the time was set for 10 minutes. After repeated centrifugation, the solids were dissolved in deionized water for testing.
1.4 Preparation of the CdTe/ZnS core-shell quantum dots
The CdTe/ZnS quantum dots were synthesized and improved according to the method of Li’s group[
To sum up, the parameters of the 10 water-soluble core-shell quantum dots are shown in Tab.1
1.5 The UV-visible absorption and the fluorescence spectra
In order to explore the influence of shell thickness, core size and shell material of the semiconductor quantum dots on UV-VIS absorption spectrum, the eight kinds of CdTe/CdS and two kinds of CdTe/ZnS semiconductor quantum dots were measured (UV-2500 UV-vis spectrometer from Shimadsu, Japan), as shown in Fig.1 and Fig.2 respectively.
Figure 1.Absorption spectra of the CdTe/CdS quantum dots
Figure 2.Absorption spectra of the CdTe/ZnS quantum dots
The fluorescence spectrum of the CdTe/CdS and the CdTe/ZnS quantum dots under the excitation wavelength of 400 nm were obtained using the LS55 fluorescence spectrometer from Perkin Elmer, as shown in Fig.3.
Figure 3.Fluorescence spectrum of the CdTe/CdS and CdTe/ZnS quantum dots
2 Results and discussion
2.1 The influence of pH value, cladding time and material on the sample
In Tab.1, the core color was observed by sampling the CdTe at the wavelength of 365 nm (the ultraviolet light) before cladding. In the same reaction temperature, the pH value of the reaction system was changed to 9.0, 10.0, 11.0, which is corresponding to the change of the time of the reflux condensation to 2 hours, 6 hours, and 10 hours. The preparation of the CdTe core was observed under the ultraviolet light at the wavelength of 365 nm, presenting green, yellow, and orange colors. The different luminescent colors indicate the different core sizes. Tab.1 shows that the core sizes increased gradually from the colors of green, yellow to orange. The overall sizes of the quantum dots in Tab.1 were estimated according to the luminescent colors.
Eight samples were the water-soluble TGA-CdTe/CdS colloidal quantum dots. The core of S1-S3 radiated orange color under the ultraviolet irradiation, the cladding time was 30 min, 1 h, and 2 h respectively. The core of S4-S5 radiated yellow color under the ultraviolet irradiation, the cladding time was 30 min and 1 h respectively. The core of S6-S8 radiated green color under the ultraviolet irradiation, the cladding time was 30 min, 1 h and 2 h respectively. Other two samples were the water-soluble TGA-CdTe/ZnS colloidal quantum dots. The core of S9-S10 radiated orange color under the ultraviolet irradiation, the cladding time was 30 min, 1 h respectively.
To be specific, it is found that the experimental results of the S6-S8 samples indicate that different cladding time causes the change of the overall size of quantum dots under the same color of the core. The overall size of quantum dots increases with the increase of the cladding time, such as the CdTe/CdS and the CdTe/ZnS core-shell quantum dots under the same core colors of orange. And the cladding time is different for 30 min and 1 h. For example, comparing the experimental results of S9 and S10 to those of S1 and S2, it is shown that the size of the CdTe/ZnS quantum dots is larger than the corresponding of the CdTe/CdS sample.
2.2 The influence of the cladding and production time of the core on the UV-vis absorption spectrum
Figure 1 illustrates the first absorption peak of position for the CdTe/CdS quantum dots. Obviously, taking the derivative of a curve, the position with the highest slope is the absorption peak. The first absorption peaks of S1-S8 were 550 nm, 555 nm, 560 nm, 540 nm, 545 nm, 510 nm, 515 nm, and 525 nm, respectively. Figure 1 shows that the quantum dots size is increased and the absorption peak is redshifts by the increase of the cladding time under the same case of the core. Similarly, in the case of the same cladding time, the size of quantum dots is increased and the absorption peak is redshifts by the increase of the core fabrication time. It is observed that the first absorption peak of S9-S10 samples is 570 nm and 580 nm in Fig.2. With the increase of the cladding time, the size of the quantum dots increases, and the absorption peak of the CdTe/ZnS quantum dots are also redshifts.
Yu’s team[
where, D(nm) is the size of quantum dots, and λ(nm) is the wavelength of the first excitonic absorption peak of the corresponding sample.
The properties of the core-shell quantum dots make them different from the bulk materials or atoms and molecules. As the size of the quantum dots decreases, the quantum confinement effect is enhanced. The bandgap of the semiconductor follows Eq.(2)[
where, E(r) is the absorption bandgap of the material, Egis the bandgap of the material, R is the particle radius, the second term is the quantum confined energy (blue shift), the third term is the Coulomb potential energy of the electron-hole pair (red shift), and the fourth term is the effective Rydberg energy.
The second term in the formula is much larger than the third and fourth terms, so that the quantum dots diameter (2R) increases and the E(r) decreases, the corresponding absorption and the luminescence spectra generate redshift.
In summary, with the increase of quantum dots size, the absorption peak is redshift; on the contrary, if the size decreases, the absorption peak will be blue shift.
2.3 Fluorescence characteristics of CdTe/CdS and CdTe/ZnS core-shell quantum dots
Figure 3 shows the fluorescence spectra are at the same core (CdTe) when the shell thickness increases to a certain degree (cladding time 30 min-1 h). The fluorescence intensity increases to the peak. At this time, if the shell thickness is continuously increased, the fluorescence intensity will decrease (e.g. S6, S7, S8). The fluorescence intensity also decreases when the core size is increased (e.g. S6, S4, S1), and the fluorescence spectrum is broadened. Some large cores growth—the so-called Ostwald ripening, causing the size distribution broadening and a wide emission line width. Some types of gradient core-shell structures are known for suppressing Auger recombination and possess high PL[
The broadening is due to the conversion of the CdTe/CdS quantum dots from type I to type II[
With the increase of the core size, the potential energy at the conduction band bottom of the core increases, while the potential energy at the conduction band bottom of the shell is relatively low, and the probability of electron distribution in the shell also increases. At this time, the potential energy difference between the conduction band bottom and the valence band top is decreased, so the absorption and the luminescence peaks have redshift accordingly[
3 Conclusion
Firstly, 10 kinds of CdTe/CdS and CdTe/ZnS core-shell quantum dots with different core sizes and shell thicknesses were synthesized by aqueous phase. In this work, the production method of the CdTe core was improved and the high quality of the NaHTe sample was obtained. The experimental results show that the higher the pH value, the faster the reaction speed; but the lower the UV luminescence intensity.
The uv-vis absorption spectrum demonstrates that the size of quantum dots is increased and the absorption peak is redshift in the same core with the increase of the cladding time; similarly, with the increase of the core fabrication time, the absorption peak is also redshift in the case of the same cladding time. In fluorescence spectrum experiments, the CdTe/CdS quantum dots convert between type I and type II according to the different core and shell sizes of quantum dots, resulting in the different fluorescence lifetime and intensity. When the thickness of the CdTe/ZnS shell increases, the fluorescence peak appears redshift. This is because the shell thickness of ZnS reduces the number of dangling bonds and surface defect states on the core surface, which increases the probability of electron hole pairs recombination, thus increasing the fluorescence peak.
References
[1] Gulin Alexander, Shakhov Aleksander, Vasin Alexander, et al. ToF-SIMS depth profiling of nanoparticles: Chemical structure of core-shell quantum dots. Applied Surface Science, 481, 144-150(2019).
[2] Min Chan-Hong, Joo Jin. Studies on the effect of acetate ions on the optical properties of InP/ZnSeS core/shell quantum dots. Journal of Industrial and Engineering Chemistry, 82, 254-260(2020).
[3] Soheyli Ehsan, Behrouzi Shima, Sharifirad Zeynab, et al. Multi-colored type-I Ag-doped ZnCdS/ZnS core/shell quantum dots with intense emission. Ceramics International, 45, 11501-11507(2019).
[4] Xu Ning, Piao Mingxing, Arkin Kamile, et al. Imaging of water soluble CdTe/CdS core-shell quantum dots in inhibiting multidrug resistance of cancer cells. Talanta, 201, 309-316(2019).
[5] T Shelawati, M S Nurisya, Tim C Kar, et al. Effects of step-potential on confinement strength of strain-induced type-I core–shell quantum dots. Superlattices and Microstructures, 131, 95-103(2019).
[6] R Hamood, El-sadek M S Abd, A Gadalla. Facile synthesis, structural, electrical and dielectric properties of CdSe/CdS core-shell quantum dots. Vacuum, 157, 291-298(2018).
[7] Jing Liang, Liangliang Zhou, Bin Li, et al. Research on the preparation, structure and infrared properties of Sb2Te3 quantum dots. Infrared and Laser Engineering, 49, 0103002(2020).
[8] Liju Tan, Hailu Fu, Tian Shi, et al. Molecularly imprinted based on CdTe quantum dots for the determination of sulfonamide residues in water. Optics and Precision Engineering, 26, 2253-2260(2018).
[9] Joo Jinwhan, Choi Yonghoon, Suh Yo-Han, et al. Synthesis and characterization of In1−xGaxP@ZnS alloy core-shell type colloidal quantum dots. Journal of Industrial and Engineering Chemistry, 88, 106-110(2020).
[10] Zhiming Wang, Dong Zhou, Qi Guo, et al. Study on the mechanism of dark current degradation of HgCdTe photovoltaic devices induced by γ-irradiation. Infrared and Laser Engineering, 48, 0916001(2019).
[11] Q Wang, Y Kuo, Y Wang, et al. Luminescent properties of water-soluble denatured bovine serum albumin-coated CdTe quantum dots. Journal of Physical Chemistry B, 110, 16860-16866(2006).
[12] Zhu Jian, Wang Si-Nan, Li Jian-Jun, et al. The effect of core size on the fluorescence emission properties of CdTe@CdS core@shell quantum dots. Journal of Luminescence, 199, 216-224(2018).
[13] L Li, C Yu, Y Ding, et al. Synthesis of functionalized core–shell CdTe/ZnS nanoparticles and their application as a fluorescence probe for norfloxacin determination. European Journal of Inorganic Chemistry, 2013, 2564-2570(2013).
[14] W W Yu, L Qu, W Guo, et al. Experimental determination of the extinction coefficient of CdTe, CdSe, and CdS nanocrystals. Chemistry of Materials, 15, 2854-2860(2004).
[15] Y Kayanuma. Quantum-size effects of interacting electrons and holes in semiconductor microcrystals with spherical shape. Physical Review B Condensed Matter, 38, 9797-9805(1988).
[16] X Jin, K Xie, T Zhang, et al. Cation exchange assisted synthesis of ZnCdSe/ZnSe quantum dots with narrow emission line widths and near-unity photoluminescence quantum yields. Chemical Communications, 56, 6130-6133(2020).
[17] Park Young-Shin, Lim Jaehoon, I Klimov Victor. Asymmetrically strained quantum dots with non-fluctuating single-dot emission spectra and subthermal room-temperature linewidths. Nature Materials, 18, 249-255(2019).
[18] Q Zeng, X Kong, Y Sun, et al. Synthesis and optical properties of type II CdTe/CdS core/shell quantum dots in aqueous solution via successive ion layer adsorption and reaction. Journal of Physical Chemistry C, 112, 8587-8593(2008).
[19] J M Tsay, M Pflughoefft, L A Bentolila, et al. Hybrid approach to the synthesis of highly luminescent CdTe/ZnS and CdHgTe/ZnS nanocrystals. Journal of the American Chemical Society, 126, 1926-1927(2004).
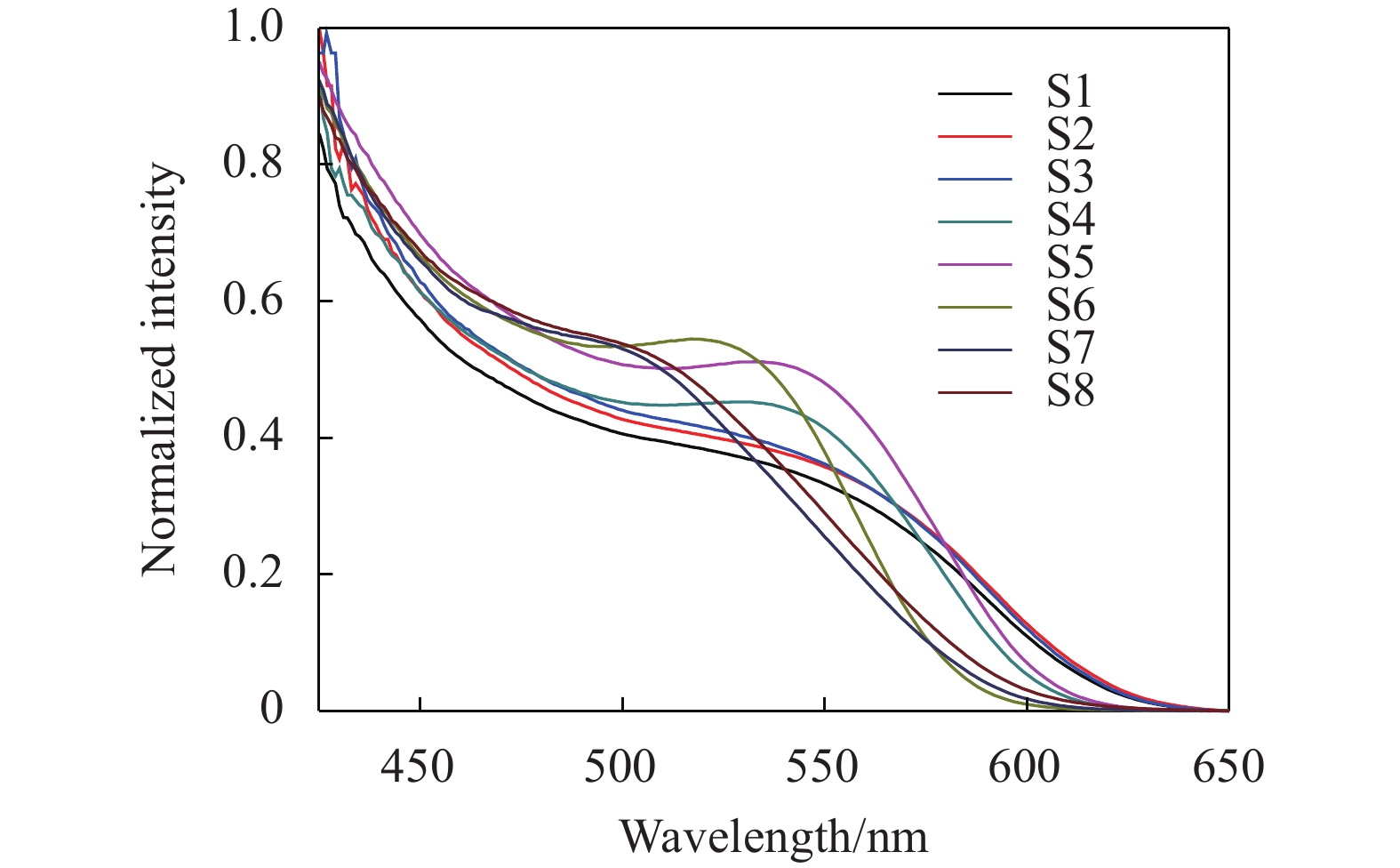
Set citation alerts for the article
Please enter your email address