
- Journal of Inorganic Materials
- Vol. 35, Issue 1, 139 (2020)
Abstract
There is a growing desire to develop renewable and clean energy, due to environmental problems such as pollution caused by fossil fuels[
In recent years, some advancements have been made on the anode materials of Na ion battery. Like metal tin has been proved as high specific capacity anode material, but impeded its practical application due to huge volume change during its working process[
In the present study, we reported the intercalation of transition metal ions of manganese into layered V2C MXene by ion exchange method, labeled as V2C@Mn. Mn2+ was successfully decorated into the interlayer of V2C MXene and formed V-O-Mn bond according to the analysis of XRD, XAS, TEM and Element Mapping characterizations. It was demonstrated that the V2C@Mn electrodes exhibited high specific capacity and outstanding cycling performance for SIB.
1 Experimental
1.1 Synthesis of V2C and V2C@Mn MXenes
As discussed in our previous articles, V2C MXene was synthesized through selectively etching V2AlC MAX powders, which were synthesized by calcining the ball-milled V, Al and C powers under 1500 ℃ in argon atmosphere. To obtain K+ intercalated V2C (V2C@K) MXene, about 500 mg as-prepared V2C powers were immersed in 20 mL 0.2 mol/L KOH aqueous solution followed by stirring for 2 d at 45 ℃. Afterwards, the solution was centrifuged by using deionized water and ethanol for several times. Then V2C@K was obtained after freeze-drying overnight. V2C@Mn was prepared by soaking V2C@K in 0.2 mol/L Mn(NO3)2 through ion exchange between manganese ions and K+.
1.2 Material characterization
The morphology of V2C@Mn was presented with a field emission SEM (SU8220) and a TEM (JEOl JEM2010), elemental mapping images were also carried out with SEM (SU8220). X-ray diffraction (XRD) patterns were measured by a Sample horizontal high power X-ray diffractometer (Rigaku TTRIII) with Cu Kα radiation (λ=0.154 nm). Chemical compositions of V2C@Mn were analyzed by high-resolution XPS recorded with a Synchrotron Radiation Photoelectron Spectrometer Station Equipment in Hefei by using Mg Kα X-ray (hν=1253 eV). V L-edge and O K-edge XANES measurements were performed at the beamline BL12B-a (CMD) in Hefei Synchrotron Radiation Equipment. The Mn K-edge XAFS measurement was performed in the fluorescence mode at the beamline 1W1B in Beijing Synchrotron Radiation Facility (BSRF).
1.3 Electrochemical measurements
The working electrodes of V2C@Mn and V2C MXenes were prepared by mixing V2C@Mn or V2C MXene (70%), acetylene black (ACET 20%), and polyvinylidene fluoride (PVDF, 10%). Then it was coated on a copper foil after uniformly grinding in N-methyl-2-pyrrolidone (NMP) and dried in vacuum oven overnight. The batteries were assembled in an Ar filled glove box (MBraun, Germany) into coin cells (CR2032) with sodium tablets as the counter electrodes, glass fiber as the separators and 1 mol/L NaClO4 in (EC: DMC, vs. 1 : 1) as the electrolyte. Rate capability and cycle ability were achieved on a Land CT2001A cell test system at 25 ℃ in the potential range between 0.01 and 3 V. Meanwhile, cyclic voltammetry (CV) at scan rate from 0.1 to 1.0 mV·s-1 in the same voltage range was performed on the CHI660D electrochemical workstation.
2 Results and discussion
2.1 Structure characterization
V2C powers were produced by selectively etching out the Al atomic layer from the MAX phase. During etching, the surface would be functionalized with hydroxyl, oxygen or fluorine terminations, so it has a formulation V2CTx (Tx= F, O, OH). Transition metal ions of manganese entered the vanadium carbide layers by replacing the potassium ions which were intercalated into V2C MXene because of the etching effect of KOH. Manganese ions could shore up the layers of V2C MXenes during the charge and discharge process which could prevent the structural collapse and accelerate ion diffusion.
As illustrated in Fig. 1(a), the X-ray diffraction (XRD) image shows a typical (0002) peak of V2C MXene around 12° with some V2AlC residue after HF treatment. After stirring in KOH solution, the (0002) peak shifts toward lower degree, which implies the expansion of the V2C@K MXene interplaner. According to Bragg equation, the interlayer spacing of V2C@K MXene is about 0.96 nm. Interestingly, after the replacement of K+ by Mn2+, the interlayer spacing of V2C@Mn decreases to approximately 0.95 nm compared with V2C@K. This weak contraction can be attributed to greater electrostatic attraction of bivalent Mn ion than monovalent K ion. Similar shrinkage is also observed in Co, Sn intercalated MXenes[
Figure .Mophological characterization of V2C@Mn
The intercalation of manganese ions was investigated by X-ray photoelectron spectroscopy (XPS) and X-ray absorption fine spectroscopy (XAFS) as shown in Fig. 2. In the high resolution Mn2p spectrum, two predominant Mn2p3/2 peaks are located at 641.3 and 643.2 eV, corresponding to divalent manganese ions and trivalent manganese ions[
Figure .Structural characterization of V2C@Mn MXene
To study more detailed electronic structure information of V2C@Mn, V L-edge and O K-edge XANES spectra were performed (Fig. 2(d)). For the V L-edge, there are two peaks located at 518 and 525 eV, corresponding to the spin-orbit (SO) spilt L3(2p3/2) and L2(2p1/2) parts, respectively. It is obvious that the shape of two curves are very similar except that the peak position shifted to higher energy after Mn2+ intercalation. As we know, this shift refers to the elevated valence of V ions. Due to crystal field splitting, the O K-edge can divided into two parts. The first part is the region of O2p-V3d(t2g) and O2p-V3d(eg) at lower energy. The second part is the region at higher energy about 542 eV, associated with the mixture of O2p with V4sp[
2.2 Na ion storage performance
In order to evaluate the electrochemical properties of the Mn2+ intercalated V2C as anode material for NIB, 2032 coin-type cells with Na foil as counterparts were assembled. Fig. 3(a) shows the cyclic voltammetry (CV) graph of V2C@Mn MXene electrode at scan rate from 0.1 to 1.0 mV·s-1. At low scan rate 0.1 mV·s-1, there are a pair of wide redox peaks at approximately 1.5/1.8 V after the initial cycle. As the sweep rates increased, the redox peaks become more pronounced. More intuitively, all the CV curves display a quasi-rectangle shape, which indicates a typical pseudocapacitive behavior of V2C MXene for NIB. In order to add more persuasive insight, we calculated the b from anodic and cathodic currents. The b from anodic and cathodic currents are about 0.75 and 0.79 respectively, clearly indicating the storage mechanism is mainly pseudocapacitive behavior. Our CV result is similar to other works where V2C MXene also shows pseudo-capacitive behavior in Na+ storage[
Figure .Electrochemical performance in half-cell
To meet the demand of fast charge and release of energy storage devices, the rate and cycle performance of devices are extremely important. As shown in Fig 3(b), the V2C@Mn electrode not only delivers higher specific capacity than pure V2C electrode, but also shows better rate performance. Even under high current density of 5 A·g-1, V2C@Mn electrode can still deliver a capacity of 60 mAh·g-1, but for V2C electrode, it is only about 50 mAh·g-1. Fig. 3(c) shows the charge and discharge curves of V2C@Mn MXene at current densities from 0.05 to 5 A·g-1. There are about 425, 280, 170, 120, 86 and 56 mAh·g-1 under 0.05, 0.1, 0.5, 1, 2, 5 A·g-1, respectively. The shapes of charge and discharge curves are approximate slash and there are no platforms with the increase of current densities, which were consistent with CV results. The behavior demonstrates that the Na+ storing mechanism of V2C@Mn MXene is mainly capacitive behavior and with the current density increases, the contribution of capacitance also increases. More intuitively, the superiority of V2C@Mn MXene to V2C MXene is reflected in the cycle stability. After rate performance test, both of the two electrodes are carried on cycling test as shown in Fig. 3(d). There is still 70% of discharge capacity remained after 1200 long-term cycles for V2C@Mn electrode. In contrast, the specific capacity of pure V2C electrode decays very fast, only 20% capacity is preserved after 800 cycles. The higher specific capacity, more excellent rate performance and superior cycle stability of V2C@Mn are benefited from the intercalation of Mn2+ into V2C MXene layers which stables the structure and accelerates ion transmission.
The dynamics of intercalated Mn2+ was investigated by ex-situ X-ray photoelectron spectroscopy (XPS) at different charge and discharge voltages. As shown in Fig. 4, the intensity of the peaks corresponding to Mn2+ are weakened as the charging voltage increased while the peaks corresponding to Mn3+ increase, indicating that Mn2+ is oxidized to Mn3+ during charging process. On the contrary, the Mn3+ is reduced to Mn2+ during discharging process. It can concluded that Mn ions intercalation strategy not only stabilizes the structure of V2C MXene, but also increases the pseudo-capacity.
Figure .
3 Summary
In summary, Mn2+ intercalated V2C MXene was successfully synthesized through ion exchange method. By using XRD, element mapping, XPS, and XAFS characterization techniques, it can be proved that the intercalated Mn2+ not only enlarged the interlayer spacing of V2C MXene but also formed a V-O-Mn covalent bond in the interlayer, which were beneficial to improve the Na+ storage capacity and stabilize the structure of V2C MXene volume change. The results showed that V2C@Mn electrode can deliver a high specific capacity of 425 mAh·g-1 at current density of 0.05 A·g-1. Notably, over 70% capacity was still remaining after 1200 long-term cycles. We believe that ion intercalation can promote the highly development of MXene based Na+ storage.
References
[13] M BAK S, M QIAO R, L YANG W et al. Na-ion intercalation and charge storage mechanism in 2D vanadium carbide. Advance Energy Materials, 7, 1700959(2017).
[15] D WANG C, M CHEN S, H XIE et al. Atomic Sn4+ decorated into vanadium carbide MXene interlayers for superior lithium storage. Advance Energy Materials, 9, 1802977(2019).
[17] S KANG J, J HWANG, H KIM D et al. Soft
[22] Y GE, F PAN P, X PENG et al. Dual-confined sulfur cathodes encapsulated in MnO2 nanotubes and wrapped with PEDOT for high-performance lithium-sulfur batteries. Chinese Journal of Inorganic Chemistry, 39, 769-779(2019).
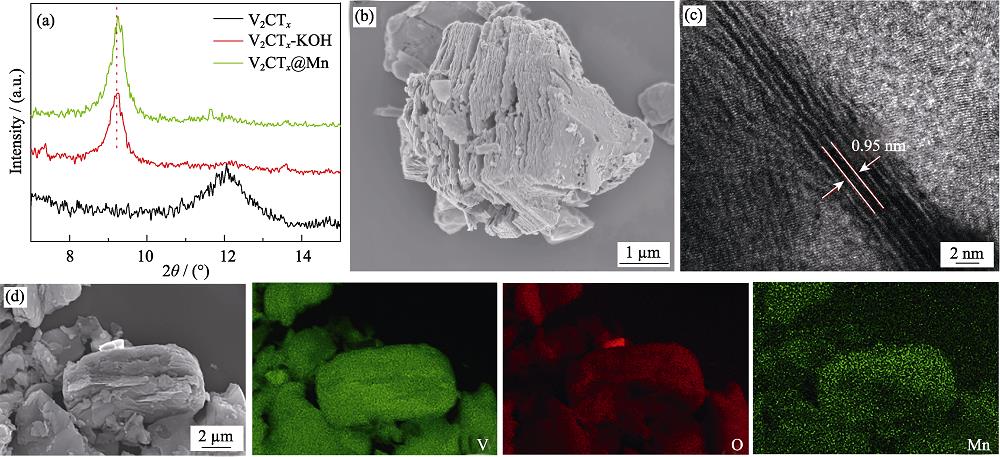
Set citation alerts for the article
Please enter your email address