Brian A. Ko, Alexei V. Sokolov, Marlan O. Scully, Zhenrong Zhang, Ho Wai Howard Lee, "Enhanced four-wave mixing process near the excitonic resonances of bulk MoS2," Photonics Res. 7, 251 (2019)

Search by keywords or author
- Photonics Research
- Vol. 7, Issue 3, 251 (2019)
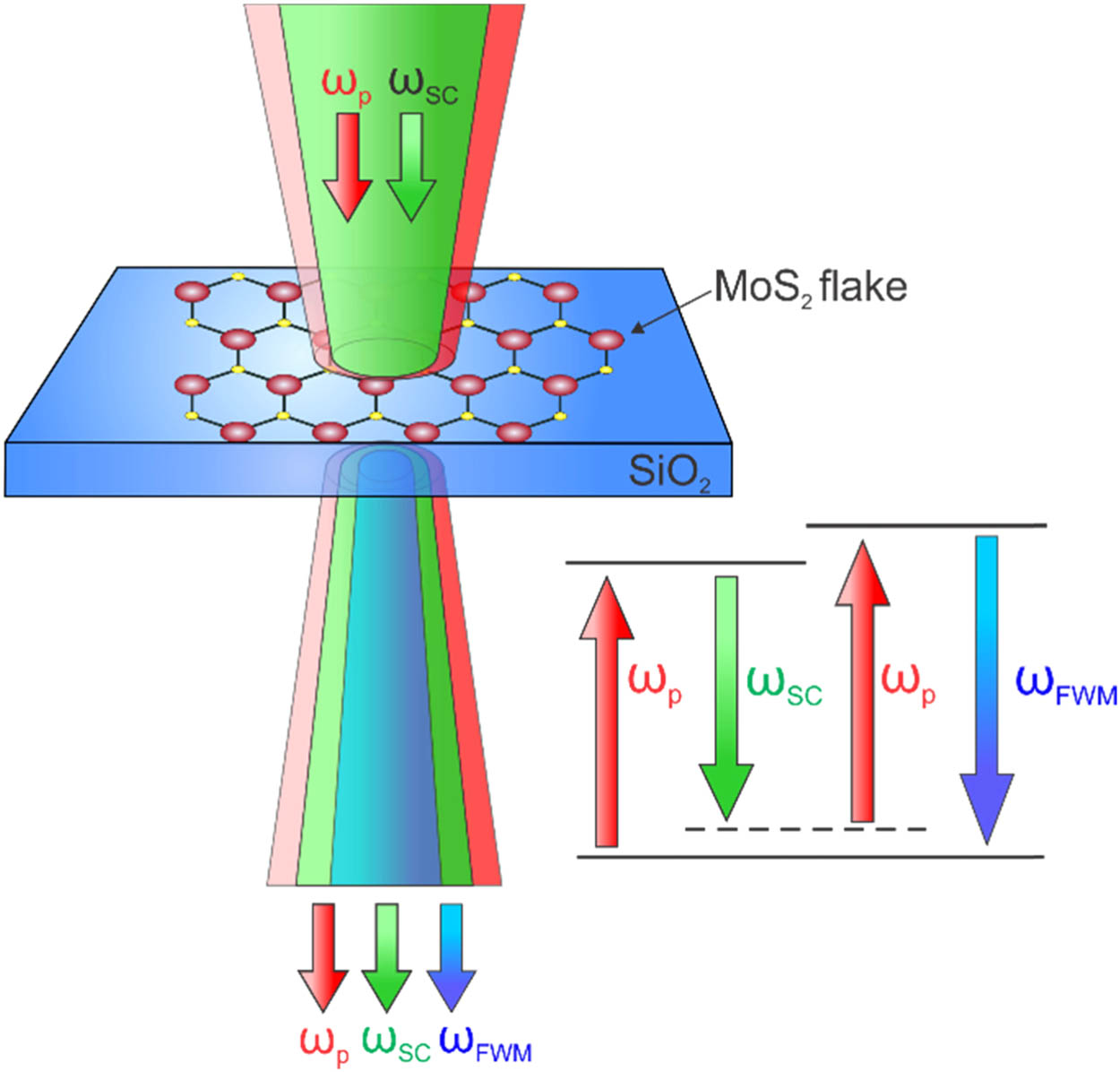
Fig. 1. Two pulses (ω p and ω SC ) are incident on an MoS 2 flake. Two photons of the pump pulse (ω p ) and one photon of the supercontinuum pulse (ω SC ) interact to produce a fourth (ω FWM ).
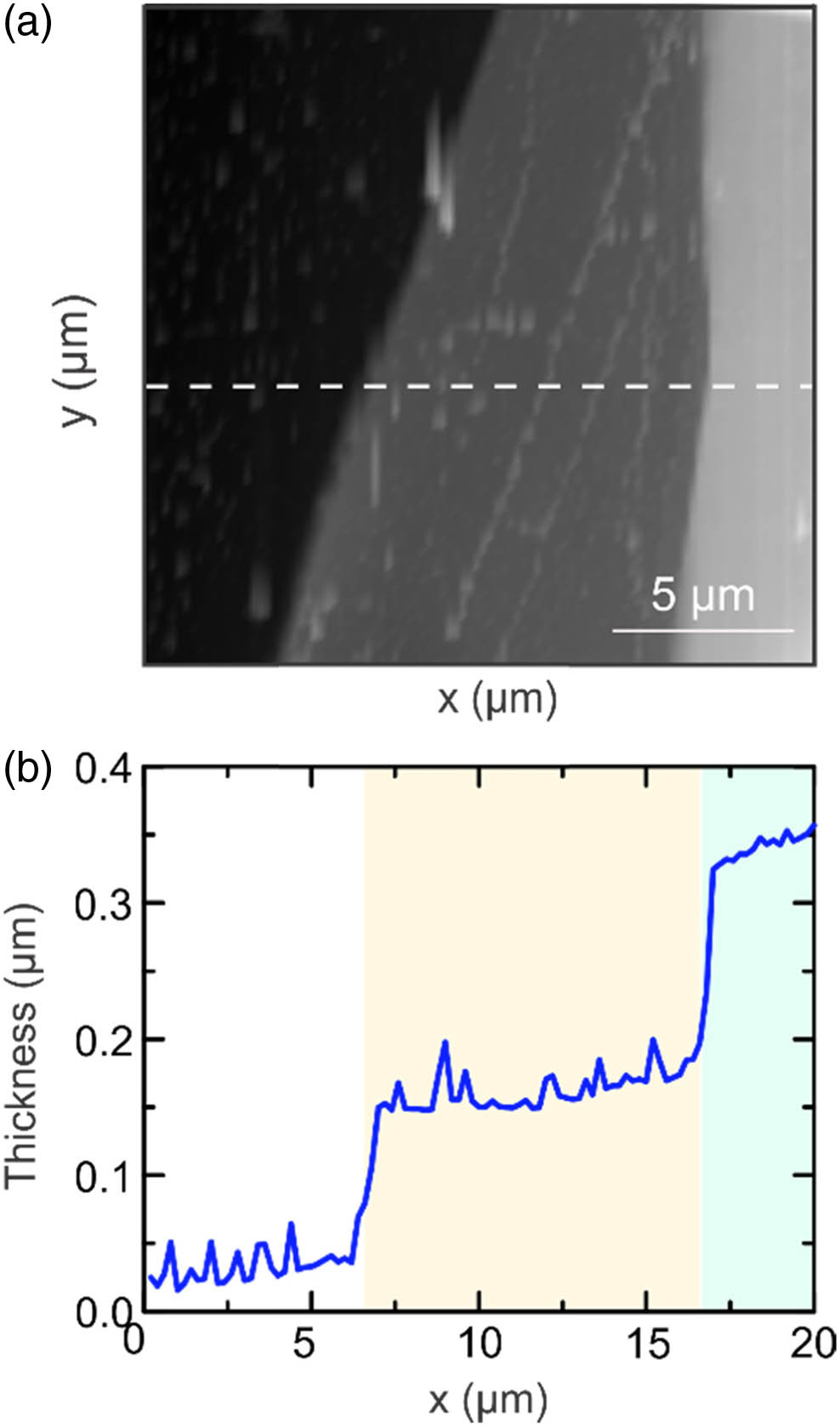
Fig. 2. (a) Atomic force microscope (AFM) image of the MoS 2 flake for the region of interest. (b) Cross section of the MoS 2 flake along the white line in (a).
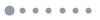
Fig. 3. Schematic of the multiplex femtosecond coherent anti-Stokes Raman spectroscopy setup. The laser provides 50 fs pulses with an 80 MHz repetition rate. RELP, razor-edge long-pass filter.
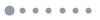
Fig. 4. (a) Optical microscope image of the MoS 2 flake. Two different locations with different thicknesses are investigated (blue, 290 nm; orange, 120 nm). FWM signal obtained from the (b) orange spot and (c) blue spot in (a). The FWM spectra are characterized by a large peak (red) with high variance in the center wavelength due to thin film interference, a more stable peak (blue) centered at approximately 680 nm from the excitonic resonances, and a small peak (green) that represents the coherent anti-Stokes Raman signal of MoS 2 .
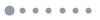
Fig. 5. Photoluminescence intensity dependence for (a) pump and (b) supercontinuum pulses on the 290 nm section of the MoS 2 flake. The quadratic dependence of the FWM signal on the pump power corresponds to requiring two pump photons for the process, while the linear dependence on the supercontinuum indicates that a single supercontinuum photon is used. Insets show the photoluminescence spectrum as pulse power is modulated. Enlarged insets can be found in Appendix C .
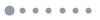
Fig. 6. Four-wave mixing spectra of the MoS 2 flake with different bandpass filters placed in the supercontinuum pulse path. The numbers indicate the central wavelength of the filters, and the pump pulse is located at wavelength of 806 nm.
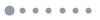
Fig. 7. (a) Optical microscope image of the MoS 2 flake. (b) Four-wave mixing spectra obtained at positions A–C marked in (a). (c) Four-wave mixing spectra obtained at positions D–F marked in (a).
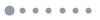
Fig. 8. Spectrum obtained at the two locations depicted in Fig. 4(a) by blocking individual pulses. Unblocked spectrum (both pulse trains) is shown in black, supercontinuum only (pump blocked) in blue, and pump only (supercontinuum blocked) in red.
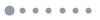
Fig. 9. Spectra (solid lines) and fitted peaks (dashed lines) of the MoS 2 flake as the power of each pulse is modulated (other pulse’s power held constant). Lorentzian peak fitting was done on each spectrum and the area of the 680 nm peak was calculated.
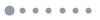
Fig. 10. Measured spectrum of the supercontinuum pulse incident on the (a) blue and (b) orange spots in Fig. 4(a) . Spectra were obtained by blocking the pump pulse and removing the short-pass filters that block the incident pulses. A neutral density filter was placed to prevent saturation while maintaining the spectral character.
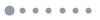
Fig. 11. B excitonic resonance of MoS 2 at the blue spot of Fig. 4(a) (thickness of 290 nm). The energy of the B exciton corresponds to a wavelength of approximately 600 nm.
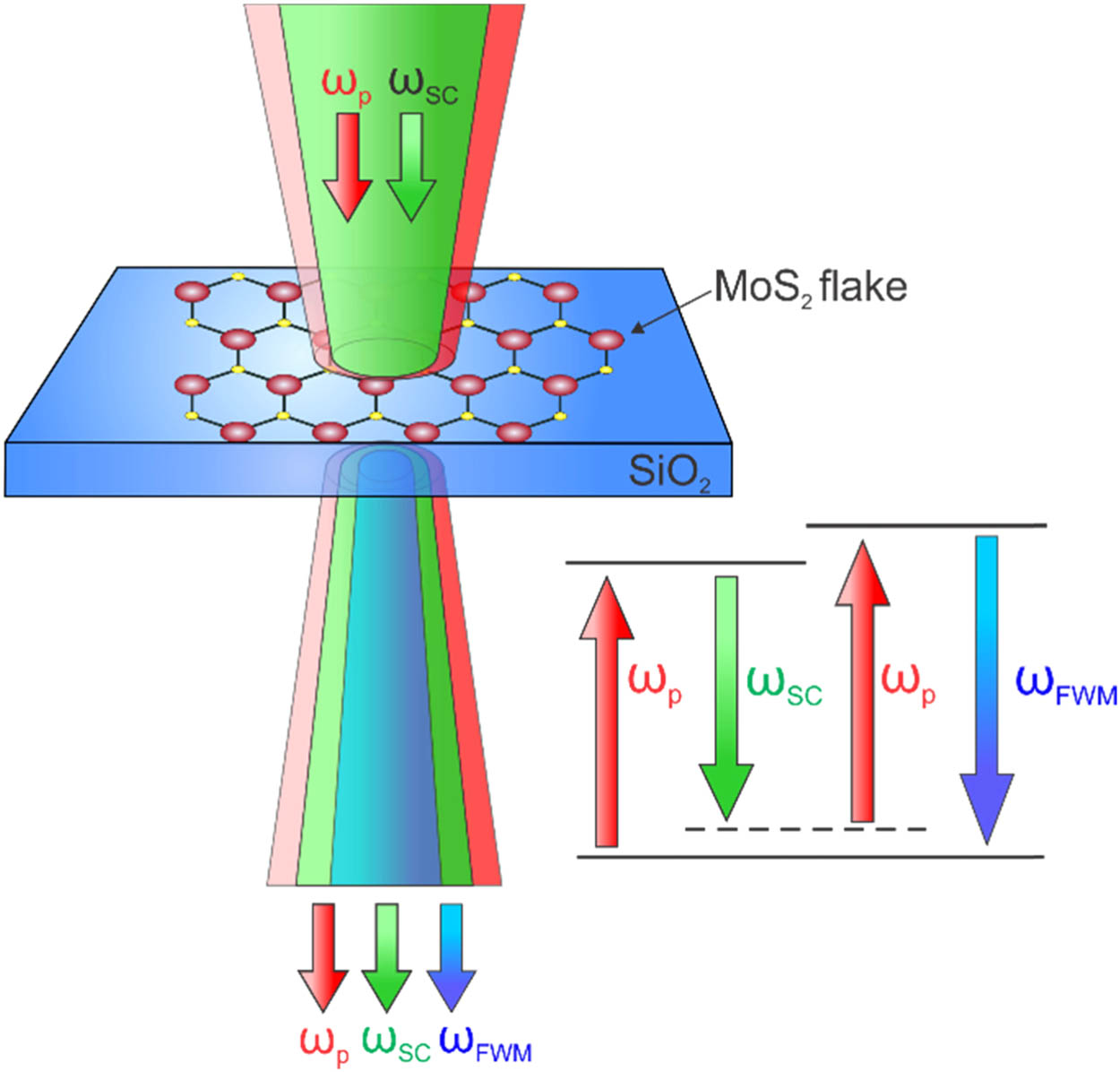
Set citation alerts for the article
Please enter your email address