Abdul Rahim, Artur Hermans, Benjamin Wohlfeil, Despoina Petousi, Bart Kuyken, Dries Van Thourhout, Roel Baets, "Taking silicon photonics modulators to a higher performance level: state-of-the-art and a review of new technologies," Adv. Photon. 3, 024003 (2021)

Search by keywords or author
- Advanced Photonics
- Vol. 3, Issue 2, 024003 (2021)
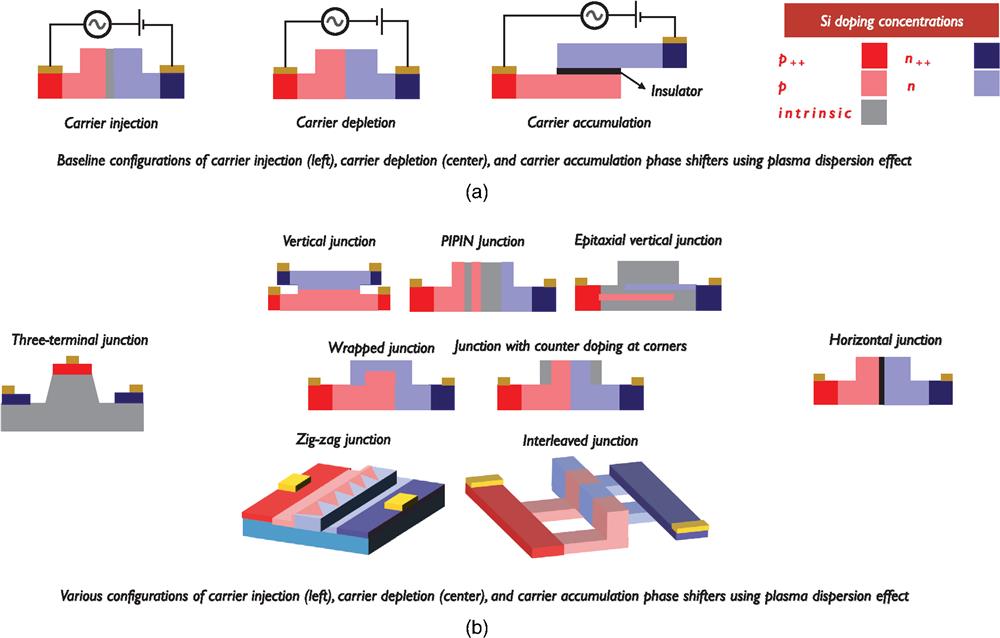
Fig. 1. (a) Baseline architecture of carrier injection, carrier depletion, and carrier accumulation plasma dispersion phase shifters. (b) Various configurations of plasma dispersion phase shifters.

Fig. 2. Representative cross sections of (a) FK-based EAMs and (b) QCS effect-based EAMs in SiPh. This figure also highlights the integration route for the respective EAMs. The term monolithic refers to the integration of a material with an SOI substrate using wafer-scale epitaxial growth resulting in PICs made out of one substrate technology in mass on a wafer level.
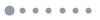
Fig. 3. Representative cross sections of (a) , (b) BTO, (c) PZT, and (d) organics modulators in SiPh. This figure also highlights the integration route for each material. The term monolithic refers to the integration of a material with a SiPh substrate using wafer-scale epitaxial growth resulting in PICs made out of one substrate technology in mass on a wafer level.
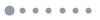
Fig. 4. Representative cross sections of (a) III–V on Si phase modulator and (b) III–V on Si EAM.
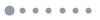
Fig. 5. Representative cross sections of (a) single-layer graphene phase/amplitude modulator and (b) double-layer graphene amplitude/phase modulator in SiPh.
|
Table 1. Prominent approaches for high-speed modulation in SiPh. The modulator implementations in SiPh use a variety of physical phenomenons, materials, optical architectures, and driver architectures.
|
Table 2. Typical and state-of-the-art performance matrix for the plasma dispersion high-speed phase modulators. The parentheses contain the best-reported result for a performance attribute. The matrix includes the results reported for O-band and C-band demonstrations.
|
Table 3. Typical and state-of-the-art performance matrix for amplitude modulation by the FK effect and QCS effect. The best-reported performance attributes are mentioned in parentheses.
|
Table 4. Typical and state-of-the-art performance matrix for the ferroelectric and organic high-speed phase modulators in SiPh.a The parentheses contain the best-reported result for a performance attribute.
|
Table 5. Typical and state-of-the-art performance matrix for the III–V on Si high-speed modulators in SiPh. The parentheses contain the best-reported result for a performance attribute.
|
Table 6. Typical and state-of-the-art performance matrix for graphene on Si high-speed modulators in SiPh. The parentheses contain the best-reported result for a performance attribute.
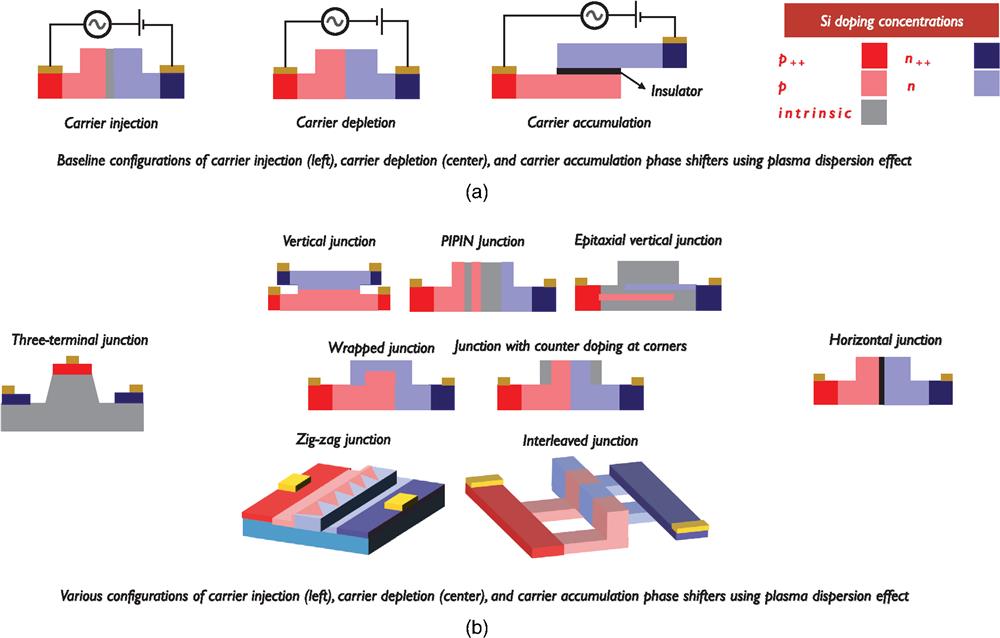
Set citation alerts for the article
Please enter your email address