S. Yu. Gus’kov, Ph. Korneev, M. Murakami. Laser-driven electrodynamic implosion of fast ions in a thin shell[J]. Matter and Radiation at Extremes, 2023, 8(5): 056602

Search by keywords or author
- Matter and Radiation at Extremes
- Vol. 8, Issue 5, 056602 (2023)
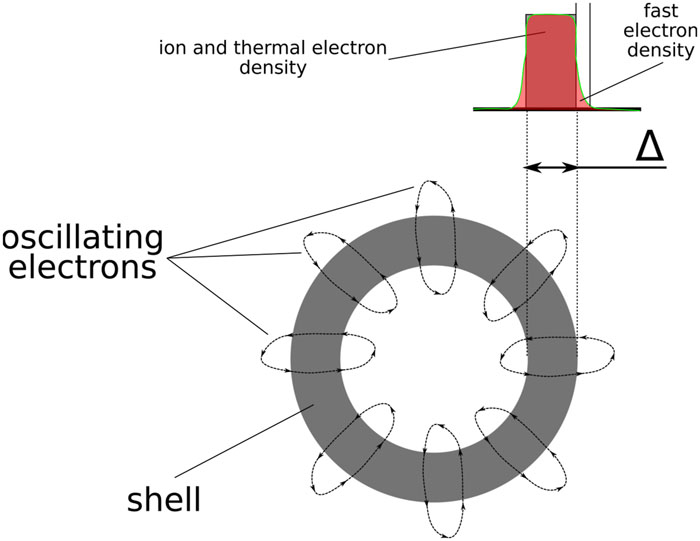
Fig. 1. Schematic illustration of the formation of a strong self-consistent potential, accelerating ions to multi-MeV energies toward the center of the shell, heated by symmetrical irradiation with short intense laser pulses. The thicknesses of the shell and Debye layer are denoted by Δ and L D , respectively.
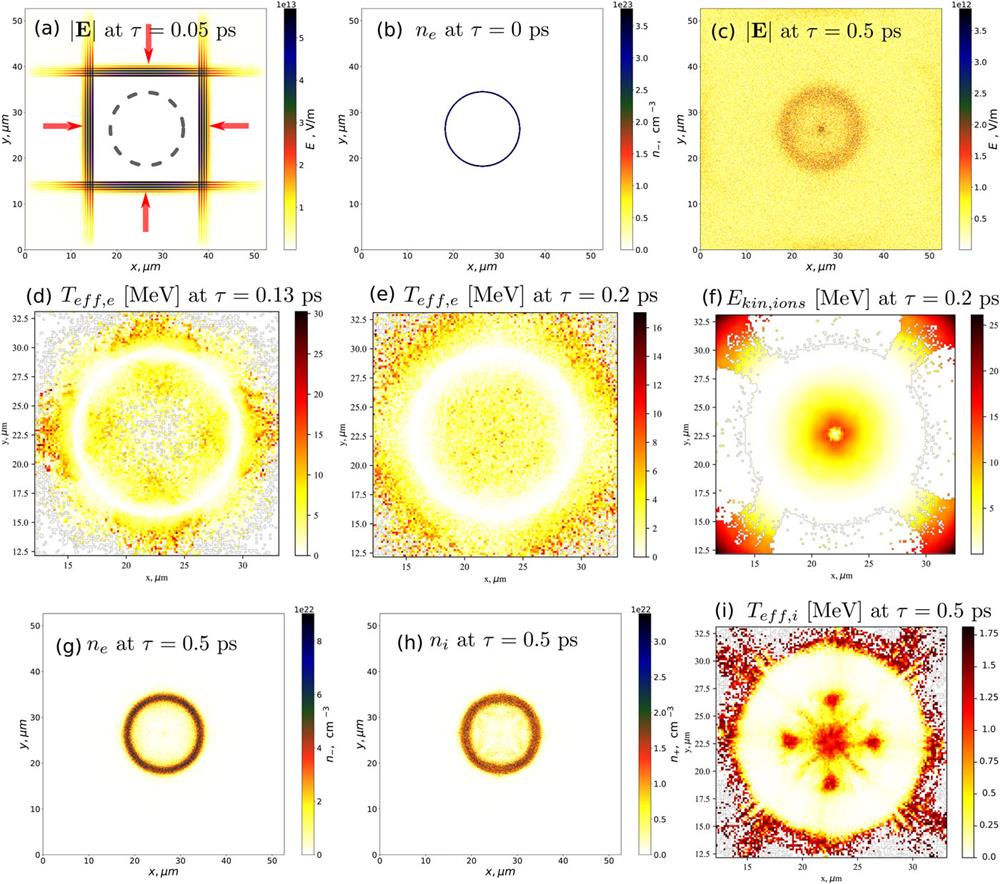
Fig. 2. Irradiation scheme and early-time results of 2D PIC simulations for the implosion of a thin plastic shell according to the bold row in Table I . (a) Irradiation scheme showing the laser pulses by the absolute value of the electric field. (b) Initial electron density. (c) Absolute value of the electric field at 0.5 ps. (d) and (e) Electron effective temperature at 0.13 and 0.2 ps, respectively. (f) Ion kinetic energy at 0.2 ps. (g) and (h) Electron and ion densities, respectively, at 0.5 ps. (i) Ion effective temperature at 0.5 ps. Note that the square-fold symmetry evident in (d), (f), and (i) is the result of the fourfold radiation geometry, shown in (a).
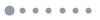
Fig. 3. Results of 2D PIC simulations of the implosion following irradiation of a thin plastic shell according to the bold row in Table I . (a), (d), (g), and (j) Electron density at times 1, 2, 4, and 5.8 ps, respectively. (b), (e), (h), and (k) Ion density at times 1, 2, 4, and 5.8 ps, respectively. (c), (f), (j), and (l) Effective ion temperature at times 1, 2, 4, and 5.8 ps respectively. The fourfold symmetry evident in (c), (f), (i), and (l) is a consequence of the fourfold irradiation scheme, shown in Fig. 2(a) .
![Proton energies just before collision [(a)–(c)] and effective temperatures just after collision [(d)–(f)] for the three cases in Table I: the first italicized row, with IL = 1019 W cm−2 [(a) and (d)]; the bold row, with IL = 1020 W cm−2 [(b) and (e)]; and the second italicized row, with IL = 1021 W cm−2 [(c) and (f)]. After the collision, the energy is transformed to the effective temperature with approximately the same value.](/Images/icon/loading.gif)
Fig. 4. Proton energies just before collision [(a)–(c)] and effective temperatures just after collision [(d)–(f)] for the three cases in Table I : the first italicized row, with I L = 1019 W cm−2 [(a) and (d)]; the bold row, with I L = 1020 W cm−2 [(b) and (e)]; and the second italicized row, with I L = 1021 W cm−2 [(c) and (f)]. After the collision, the energy is transformed to the effective temperature with approximately the same value.
|
Table 1. Parameters of matched laser pulse and shell in the range of laser intensity of the first harmonic of the Nd-laser radiation 1019–1023 W cm−2, together with characteristic values of the energy, number, and generation rate of fast ions electrodynamically imploded inside the shell at the laser energy conversion to fast-electron energy η = 0.2. Here IL is the intensity, EL is the energy, τL is the duration of one of the laser pulses, R is the radius and Δ is the thickness of the shell, εi is the estimated energy, Ni is the estimated number and Wi is the estimated flux of the imploding ions. Boldface is the main case considered and illustrated in Figs. 2 and 3 ; boldface and italic are also the cases illustrated in Fig. 4 .
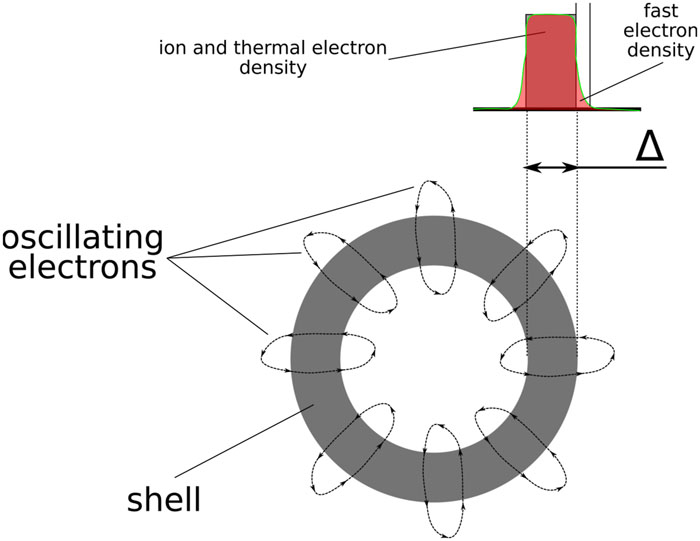
Set citation alerts for the article
Please enter your email address