Martin Divoky, Jonathan Phillips, Jan Pilar, Martin Hanus, Petr Navratil, Ondrej Denk, Tomas Paliesek, Patricie Severova, Danielle Clarke, Martin Smrz, Thomas Butcher, Chris Edwards, John Collier, Tomas Mocek, "Kilowatt-class high-energy frequency conversion to 95 J at 10 Hz at 515 nm," High Power Laser Sci. Eng. 11, 05000e65 (2023)

Search by keywords or author
- High Power Laser Science and Engineering
- Vol. 11, Issue 5, 05000e65 (2023)
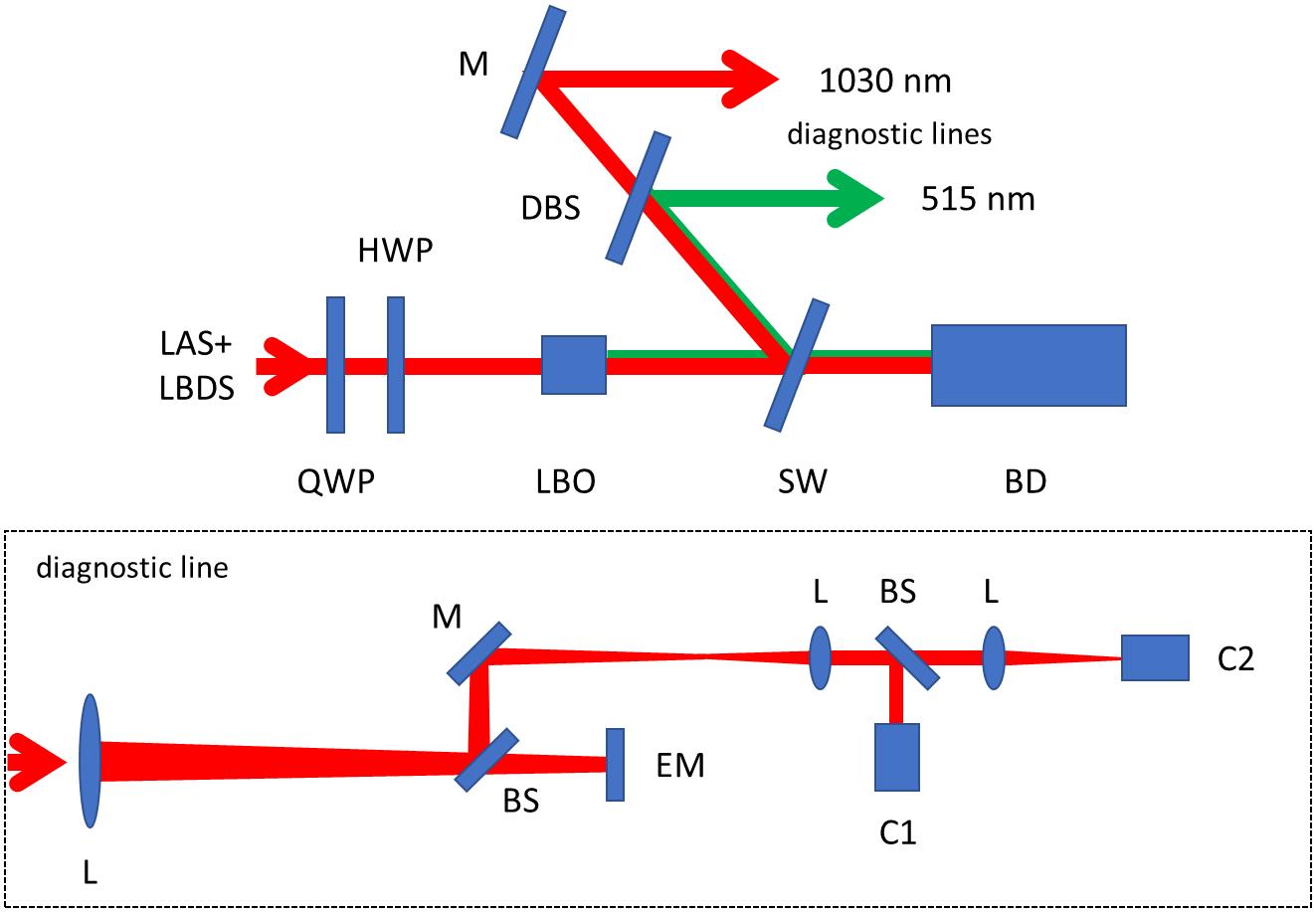
Fig. 1. Schematic layout of the conversion experiment. It consists of a laser system and laser beam distribution system (LAS+LBDS), quarter waveplate (QWP), half waveplate (HWP), conversion crystal (LBO), partially reflecting sampling wedge (SW) and beam dump (BD). The diagnostics consist of a dichroic beamsplitter (DBS), mirrors (M), lenses (L), beamsplitters (BS), an energy meter (EM), a near-field camera (C1) and a far field camera (C2). The layout of diagnostic lines is the same for both wavelengths and is shown only once.
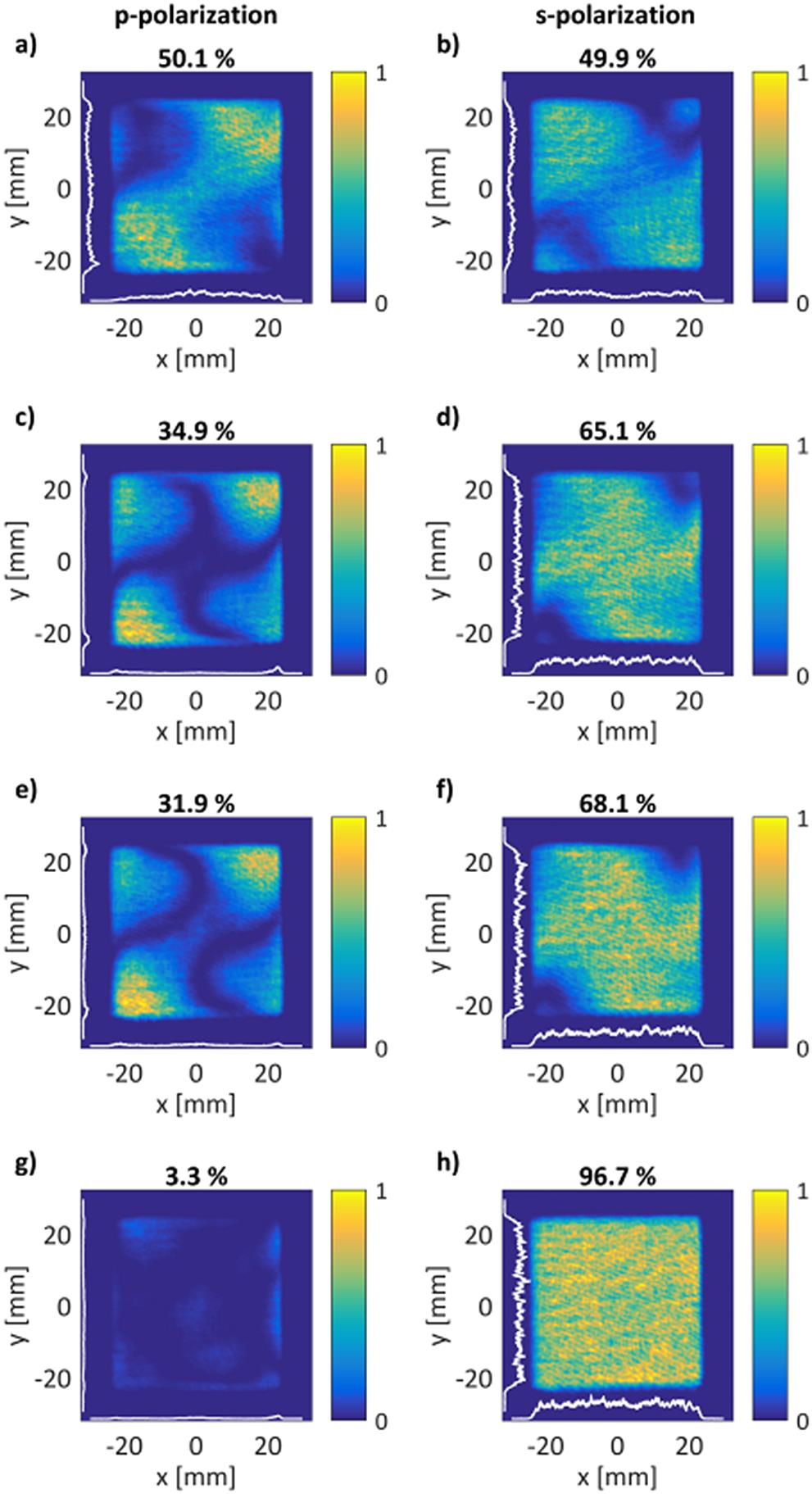
Fig. 2. Beam profiles after correction waveplates after polarizer transmitting vertical polarization (a), (c), (e), (g) or horizonal polarization (b), (d), (f), (h). (a), (b) Linear polarization entering the power amplifier with no optimization at the output. (c), (d) Optimized polarization at the input. (e), (f) Linear polarization at the input and optimized polarization at the output. (g), (h) Optimized polarization at the input and output. Beam profiles at complementary polarizations were taken under the same conditions and were normalized to the sum of both intensities. The white lines in the pictures correspond to cross-lines through the center of the beam.
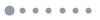
Fig. 3. Dependence of the second harmonic frequency output energy and conversion efficiency on the input energy during the energy ramp in the beginning of the experiment.
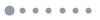
Fig. 4. Temporal evolution of the energy of the second harmonic frequency and conversion efficiency. Points where the crystal phase matching angle was optimized are marked with arrows.
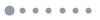
Fig. 5. Near-field beam profiles of the input beam at 1030 nm with energy of 121 J and the converted second harmonic frequency (515 nm) beam with energy of 95 J at the repetition rate of 10 Hz.
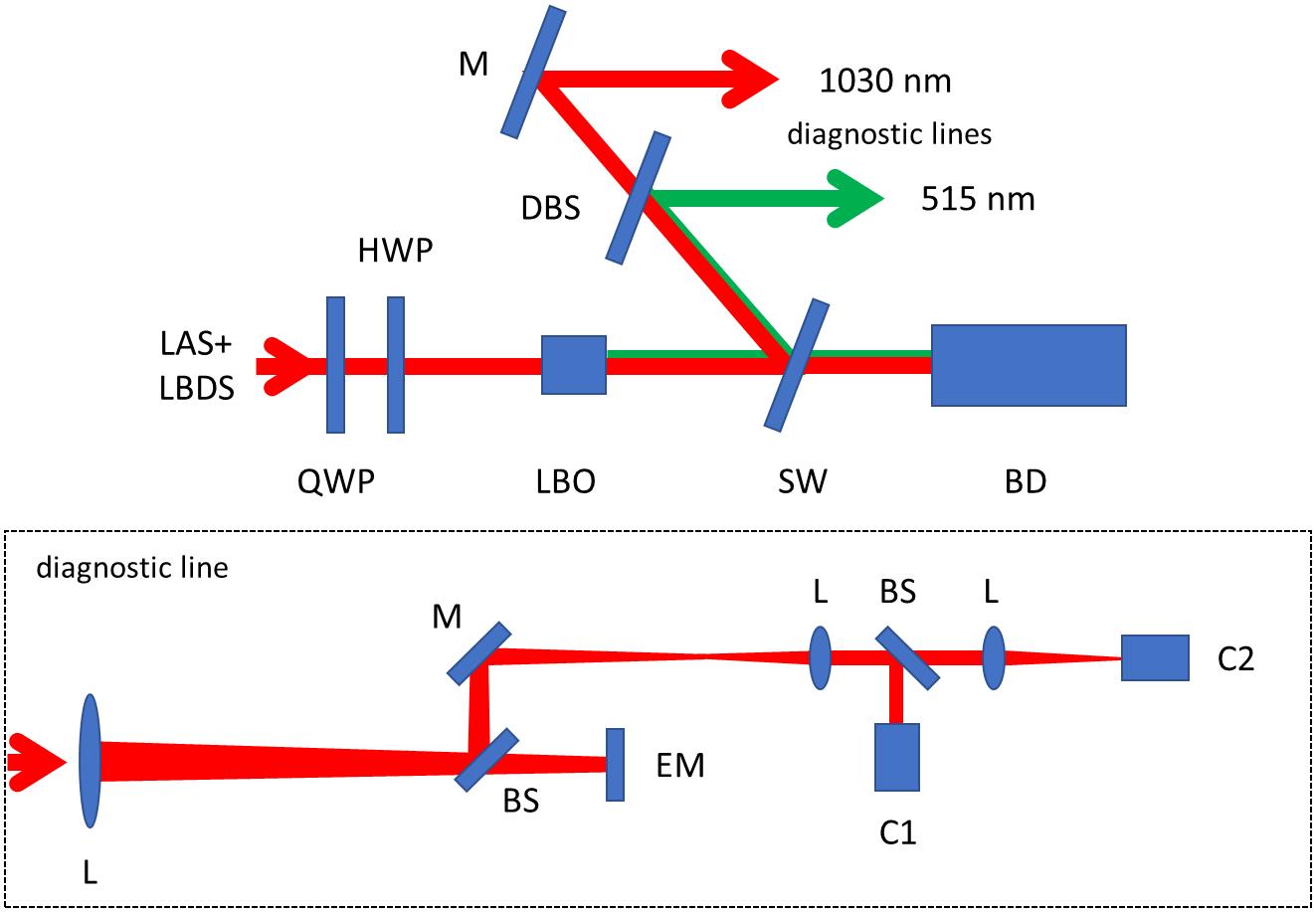
Set citation alerts for the article
Please enter your email address