Abstract
Effective detection of methamphetamine (Met) requires a fast, sensitive, and cheap testing assay. However, commercially available methods require expensive instruments and highly trained operators, which are time-consuming and labor-intensive. Herein, an antibody-modified graphene transistor assay is developed for sensitive and minute-level detection of Met in complex environments. The anti-Met probe captured charged targets within 120 s, leading to a p-doping effect near the graphene channel. The limit of detection reaches 50 aM (5.0 × 10?17 M) Met in solution. The graphene transistor would be a valuable tool for Met detection effective prevention of drug abuse.Effective detection of methamphetamine (Met) requires a fast, sensitive, and cheap testing assay. However, commercially available methods require expensive instruments and highly trained operators, which are time-consuming and labor-intensive. Herein, an antibody-modified graphene transistor assay is developed for sensitive and minute-level detection of Met in complex environments. The anti-Met probe captured charged targets within 120 s, leading to a p-doping effect near the graphene channel. The limit of detection reaches 50 aM (5.0 × 10?17 M) Met in solution. The graphene transistor would be a valuable tool for Met detection effective prevention of drug abuse.1. Introduction
It is becoming a global public health problem that drugs are frequently abused by drug addicts, athletes, and recreational users in many countries as central nervous system stimulant, becoming a global public health problem[1,2]. Among the abused illicit drugs, methamphetamine (C10H15N, molecular weight 149.24, abbreviated as Met) is the most widely used in many areas of the world[3]. It is essential to determine the concentration of stimulants in biological materials for forensic and clinical purposes. In many cases, the confirmation of stimulant abuse in body fluid is generally done by gas chromatography-mass spectrometry (GC-MS), liquid chromatography-mass spectrometry (LC-MS), high-performance liquid chromatography-mass spectrometry (HPLC-MS), capillary electrophoresis (C.E.)[4–6]. For example, Hisao reported a method to detect Met in human urine at the lowest concentrations of 3.75 ng/mL with matrix-assisted laser desorption ionization time-of-flight mass spectrometer analysis[4]. However, these analytical methods have disadvantages, such as being time-consuming and labor-intensive. In addition, the methods need expensive instruments and highly trained operators. Therefore, a rapid, ultrasensitive and easy-to-use assay is urgently required to detect Met in drug abusers' body fluid which is badly needed.
Graphene field effect transistor (GFET) device, especially graphene field effect transistor-based biosensor (GFET biosensor) device as a next-generation bioelectronics device, has become a research hotspot in microfabrication techniques[7]. GFET biosensor is particularly attractive in point-of-care testing due to lower detection limits, low cost, high sensitivity and rapid response, and reduced need for skilled personnel[8,9]. Past works have reviewed the large number of applications and possibilities that GFETs offer[10–15]. Although different types of sensors for Met detecting which are irrelevant to GFET have been developed, and still have disadvantages[4,16–18]. For instance, the cucurbit[7]uril (C.B. [7]) macrocycle as a chemical receptor to detect Met has been reported in 2017 and 2020, respectively[16,17]. Both of them show that C.B. [7] as a receptor can be highly sensitive detection of Met without screening fentanyl. However, C.B. [7] shows the highest affinity with Met and fentanyl because of the phenethyl ammonium binding epitope[19].
Here, we develop an antibody-modified GFET biosensor for the real-time monitoring of Met levels (Fig. 1). After immobilizing the Met-mAB on the graphene surface as the probe, the GFET biosensor efficiently converts Met's specific binding into the conductance changes in the graphene channel and detects Met with an LoD down to 50 aM (1 aM = 10–18 M).
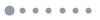
Figure 1.(Color online) (a) Schematic diagram of GFET. (b) The Image of the GFET and zoomed-in chip. (c) The fabrication process of the biosensor on graphene surface.
2. Materials and methods
2.1. Graphene synthesis and transfer process
Fig. 1 schematically shows the solution-gated graphene-FET fabrication processes. In this work, the GFET biosensor was fabricated on a high-resistance silicon substrate with 1μm thick silicon oxide[20]. Monolayer graphene was grown on Cu foils by a low pressure CVD method[21], then transferred to the SiO2/Si substrate with a ''bubble-free'' electrochemical delamination transfer[22]. The bare graphene sheet was patterned through photolithography and plasma etching to define the channel region. The FET had a channel with the length of 100μm and a width of 2 mm (W/L = 200).
2.2. Functionalization and immobilization
The anti-Met was immobilized on the graphene channel via a linker molecule 1-pyrenebutanoic acid succinimidyl ester (PASE) which attached to the graphene surface through π–π stacking (Fig. 1(c)). To functionalize anti-Met on graphene, the GFET device was immersed in a dimethyl sulfoxide solution of 5 mM PASE for 2 h at room temperature. After removing the excess PASE by rinsing with DMF and DI water several times, a polydimethylsiloxane (PDMS) open well was placed on top of the GFET device to hold the sample solutions. Then, the phosphate buffer saline (PBS) solution of 100 nM Met-mAB was dropped onto the PDMS open well and was reacted with PASE for over 6 h. The unbound Met-mAB was washed away by PBS solution. Finally, 10 mM ethanolamine was used to deactivate the excess reactive groups of PASE and avoid nonspecific binding events. The graphene with/without Met-mAB functionalization was investigated by binding energy and shown in Fig. S1. At the same time, the histogram of the variation in the initial characteristics of the transistors was shown in Fig. S2.
2.3. Characterization
The electrical properties of the GFET device were recorded by a semiconductor parameter analyzer (Keithiey B1500A). The test conditions were Ag/AgCl as standard electrodes with a source leakage voltage of 25 mV. The different concentrations of Met BSA conjugant (Met-BSA) were prepared by serially diluting the stock solution of Met-BSA. Before the electrical characterization of the device, the GFET was immersed in 100μL diluent overnight. In order to keep the same total volume (100μL), 50μL solution was taken out before an analyte solution (50μL) was injected into the PDMS open well in electrical measurements. TheIds–Vgs curve was measured to obtainVDirac after the analyte solution was injected for 20 min. The time-resolvedIds response was measured withVds = 50 mV andVgs = 0 mV. When theIds response entered saturation without significant change, an analyte solution with a higher concentration was injected.
3. Schematic design of the proposed GFET
Because PBS buffer can resist little change in acidity or alkalinity and it is isotonic with human blood, it is commonly used as a dilution of biologically active biologics. TheIds–Vg transfer characteristic curve of PBS solutions with different concentrations of Met-BSA was tested to study the influence of the concentrations of Met-BSA andVDirac, as shown inFig. 2(a). After modifying the GFET device, theVDirac is a positive offset value, meaning Met-mAB might form a p-doped effect on the graphene surface. With the concentration of Met-BSA increasing from 50 aM to 5 nM, theVDirac is a negative offset value. Precisely, the value ofVDirac shifts for a total of 14 mV. In the meantime, the value ofIds is gradually reduced by 1μA in the total test concentration of Met-BSA. With the concentration of Met-BSA increasing from 500 pM (5.0 × 10−10 M) to 5 nM, the value ofVDirac doesn't change. The result suggests that the GFET device reaches a near-saturation state at the concentration of 5 nM Met-BSA. From the results observed, the proposed mechanism and the change of Dirac cone for the Met detection by using Met-mAB based biosensor is shown inFig. 3. Briefly, the Met not only facilitates antibody binding but also accelerates electron transfer, thus amplifies electrochemical detection signal.
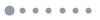
Figure 2.(Color online)Ids–Vg transfer characteristic curve in (a) PBS solution, (b) fluidartificial saliva, (c) bovine serum fluid, and (d) artificial urine.
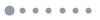
Figure 3.(Color online) (a) Schematic illustration of the detection mechanism. (b) Schematic illustration of the change of Dirac cone causing by electron doping.
In addition to the PBS buffer system, artificial urine, bovine serum fluid, and artificial saliva, commonly used as artificial body fluids, have been studied. Under the same test conditions, theIds–Vg transfer characteristic curve of artificial body fluids with different concentrations of Met-BSA was tested to study the influence of the artificial body fluids andVDirac, as shown inFig. 2. After adding the three artificial body fluids with Met-BSA at 50 aM to the GFET device, the GFET device has a clear response, and all of theVDirac is a negative offset value. With the concentration of Met-BSA in the three artificial body fluids increasing from 50 aM to 5 nM, theVDirac is also a negative offset value. In artificial saliva and bovine serum fluids, the shifted value ofVDirac is 16 and 24 mV, respectively. Furthermore, the value ofIds is reduced by about 9 and 8μA, respectively. In artificial urine, the shifted value ofVDirac is 24 mV, and about 1μA increased the value ofIds. Similarly, there is no shift after the GFET device reaches a near-saturation state at the concentration of 5 nM Met-BSA.
The real-time response of GFET to increasing Met-BSA concentrations is expressed as |(Ids–Ids,0)/Ids,0|vs. time, whereIds,0 is the drain current atVg = 0 V (Fig. 4). With the concentration of Met-BSA in the PBS buffer system increasing from 50 aM to 5 nM, the total current reduction (ΔIds(max)) is about 13% of the initial currentIds ratio (Fig. 4(a)). After adding the concentration of 50 aM Met-BSA to the PDMS well of the device, the response currentIds changes significantly within 30 s, and the ΔIds is about 20% of the ΔIds(max) (Fig. 4(b)). With the concentration of Met-BSA increasing, its response tends to be weaken. At the concentration of 5 nM Met-BSA, the GFET device test obtains a similar result as shown inFig. 4(b), in which the GFET device reaches a near-saturation state. That is because most of the monoclonal antibody Met-mAB modified on the surface of the graphene channel binds specifically to the antigen Met-BSA, resulting in no active antibody binding to the antigen.
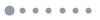
Figure 4.(Color online) GFET sensor devices in PBS solution detect Met-BSA in real time. (a)Ids–t output characteristic curve. (b) Normalized treatment. (c) Response time. (d) Schematic diagram for Met detection in biological fluids using the GFET.
As shown inFig. 4(c), the GFET device response rate to the Met-BSA in the PBS buffer system includes three processes: fast (ca. 30 s), slow (ca. 100 s), and fast (ca. 60 s). Although the GFET device shows two fast processes, the reasons behind the two processes are different. At the first fast process, the amount of antibody Met-mAB on the surface of the graphene channel is much more than that of antigen Met-BSA which can quickly bind specifically to the antibody Met-mAB within a short response time. As the antibody Met-mAB on the surface binds to antigen Met-BSA, the amount of antibody with specific binding activity decreases, the binding time increases, and the response speed becomes slower.
At the second fast process, the amount of antigen Met-BSA which is added into the PDMS well of the GFET device is much more than the residual amount of the antibody Met-mAB. The antigen Met-BSA can quickly bind to the residual antibody Met-mAB, and the response time is shortened again. Therefore, in view of the above results, the antibody-modified GFET assay has a potential application for Met detection in biological fluids (Fig. 4(d)).
4. Conclusion
In this work, a liquid-gated GFET biosensor based on the antigen-antibody specificity binding is fabricated successfully. Not only can the GEFT biosensor have detection limits as low as 50 aM, which is much lower than the currently reported detection methods, but the detection speed is fast, and it takes only about one minute to complete the detection. The GFET biosensor exploring fast and sensitive properties has opened up a bright prospect for the medical diagnostics. Although the GFET biosensor has the characteristics of fast and efficient detection, the quantitative relationship between Met-BSA antigen concentration and electrical response signals needs to be further studied.
Appendix A. Supplementary materials
Supplementary materials to this article can be found online athttps://doi.org/10.1088/1674-4926/44/2/022001.
References
[1] T C Chang, C Y Chiang, M H Lin et al. Fiber optic particle plasmon resonance immunosensor for rapid and sensitive detection of methamphetamine based on competitive inhibition. Microchem J, 157, 105026(2020).
[2] A Kumar, I Dangi, R Pawar. Drug addiction: A big challenge for youth and children's. Int J Res Pharm Pharm Sci Internet, 4, 35(2019).
[3] M Harastani, A Benterkia, F M Zadeh et al. Methamphetamine drug abuse and addiction: Effects on face asymmetry. Comput Biol Med, 116, 103475(2020).
[4] K R Wu, H H Hsiao. Rapid and accurate quantification of amphetamine and methamphetamine in human urine by antibody decorated magnetite nanoparticles coupled with matrix-assisted laser desorption ionization time-of-flight mass spectrometer analysis. Anal Chimica Acta, 1025, 134(2018).
[5] G Bor, U Bulut, E Man et al. Synthetic antibodies for methamphetamine analysis: Design of high affinity aptamers and their use in electrochemical biosensors. J Electroanal Chem, 921, 116686(2022).
[6] F Ghorbanizamani et al. Ionic liquid-hydrogel hybrid material for enhanced electron transfer and sensitivity towards electrochemical detection of methamphetamine. J Mol Liq, 361, 119627(2022).
[7] C H Dai, Y Q Liu, D C Wei. Two-dimensional field-effect transistor sensors: The Road toward commercialization. Chem Rev, 122, 10319(2022).
[8] H Y Abbasi, Z Tehrani, A Devadoss et al. Graphene based electrochemical immunosensor for the ultra-sensitive label free detection of Alzheimer's beta amyloid peptides Aβ(1-42). Nanoscale Adv, 3, 2295(2021).
[9] R R Wang, Y Mao, L Wang et al. Solution-gated graphene transistor based sensor for histamine detection with gold nanoparticles decorated graphene and multi-walled carbon nanotube functionalized gate electrodes. Food Chem, 347, 128980(2021).
[10] H Kang, X J Wang, M Q Guo et al. Ultrasensitive detection of SARS-CoV-2 antibody by graphene field-effect transistors. Nano Lett, 21, 7897(2021).
[11] R Forsyth, A Devadoss, O J Guy. Graphene field effect transistors for biomedical applications: Current status and future prospects. Diagnostics, 7, 45(2017).
[12] W Fu, M El Abbassi, T Hasler et al. Electrolyte gate dependent high-frequency measurement of graphene field-effect transistor for sensing applications. Appl Phys Lett, 104, 013102(2014).
[13] B B Zhan, C Li, J Yang et al. Graphene field-effect transistor and its application for electronic sensing. Small, 10, 4042(2014).
[14] A Nag, A Mitra, S C Mukhopadhyay. Graphene and its sensor-based applications: A review. Sens Actuat A, 270, 177(2018).
[15] L Q Wang, X J Wang, Y G Wu et al. Rapid and ultrasensitive electromechanical detection of ions, biomolecules and SARS-CoV-2 RNA in unamplified samples. Nat Biomed Eng, 6, 276(2022).
[16] M Jang Y Jang, H Kim et al. Point-of-use detection of amphetamine-type stimulants with host-molecule-functionalized organic transistors. Chem, 3, 641(2017).
[17] X C Du, H X Hao, A J Qin et al. Highly sensitive chemosensor for detection of methamphetamine by the combination of AIE luminogen and cucurbit[7]uril. Dyes Pigments, 180, 108413(2020).
[18] S Mandani, B Rezaei, A A Ensafi. Sensitive imprinted optical sensor based on mesoporous structure and green nanoparticles for the detection of methamphetamine in plasma and urine. Spectrochim Acta A, 231, 118077(2020).
[19] S Ganapati, L Isaacs. Acyclic cucurbit[n]uril-type receptors: Preparation, molecular recognition properties and biological applications. Isr J Chem, 58, 250(2018).
[20] C H Dai, M Q Guo, Y L Wu et al. Ultraprecise antigen 10-in-1 pool testing by multiantibodies transistor assay. J Am Chem Soc, 143, 19794(2021).
[21] Y B Yang, X D Yang, X M Zou et al. Ultrafine graphene nanomesh with large on/off ratio for high-performance flexible biosensors. Adv Funct Mater, 27, 1604096(2017).
[22] Y Q Song, W T Zou, Q Lu et al. Graphene transfer: Paving the road for applications of chemical vapor deposition graphene. Small, 17, 2007600(2021).