Abstract
We report on the performance of a continuous-wave laser in-band diode-pumped at 912 nm with high output power and excellent beam quality. The laser produced an output power of 19.8 W at 1063 nm with an optical efficiency of 59.3% and slope efficiency of 62.7%. The laser threshold was of the absorbed pump power, and laser output beam quality was in the horizontal and vertical directions. The strength of thermal lensing at full output power (33.4 W of absorbed power) was measured to be an average of 8.6 diopters. It is shown that thermal lensing is reduced by a factor of 2 with respect to the lasers, thus opening a way for further output-power scaling.1. INTRODUCTION
Diode end-pumped solid-state lasers with high efficiency, excellent beam quality, and high output powers are of great interest due to their applications in areas such as micromachining and nonlinear optics. High-power operation of lasers, however, is usually limited by detrimental thermal lensing effects. Thermal lensing affects the stability of a resonator, degrades the output beam quality, and, in extreme cases, can result in mechanical failure of the crystal. The strength of thermal lensing is usually governed by the thermal properties of the gain medium as well as the amount of heat it generates [1]. One way to overcome thermal lensing problems is to reduce the source of heating within the gain medium. This was extensively investigated with the well-known crystal. Nd-doped solid-state lasers such as and are conventionally pumped at 808 nm, which usually leads to strong thermal lensing effects at high output powers. In order to reduce the thermal issues and scale up the output power, an efficient pumping method was presented which pumps the laser at absorption lines with longer wavelengths (such as 880, 888, and 914 nm) than the traditional 808 nm [2–13]. Pumping at longer wavelengths can result in a significant reduction in quantum defect (energy difference between the absorbed pump and laser output photons). For example, pumping of a 1064 nm laser at 914 instead of 808 nm results in the reduction of fractional thermal loading [] from 24% to 14%. This means that a lower amount of pump energy would be converted into heat. The detrimental thermal effects would thus be pushed to the higher levels of the absorbed pump power and, as a result, a higher output power can be obtained. In addition to reduction of thermal lensing, a lower quantum defect enhances the theoretical upper limit of slope efficiency of a laser system. This was demonstrated in lasers, where slope efficiencies of up to 80.7% in the continuous-wave (CW) [3] and up to 77.1% in the mode-locked regimes [14] were reported using in-band diode pumping at 914 nm (maximum output powers were 11.5 and 6.7 W, respectively). In addition, it was also shown that the strength of thermal lensing with 914 nm pumping is more than two times weaker than that with traditional 808 nm [12]. Therefore, power scaling of the Nd-doped lasers based on in-band pumping around 900 nm is a promising alternative. Recently, a 33.8 W CW laser with optical-to-optical efficiency of 50% and threshold pump power of was also realized with 914 nm pumping [15].
As mentioned, the reduction of heating was extensively studied with crystal since it suffers from moderate thermal conductivity ( [1]). This will eventually limit power scaling of lasers based on this crystal. Ultimately, a laser crystal with high thermal conductivity and excellent laser properties is needed. An attractive solution to this issue is to use the crystal of , which has high thermal conductivity ( [16]) and similar laser properties to those of .
Despite notable research on lasers pumped around 914 nm [3,4,12,14,15], there is only one proof-of-principle work on CW lasers using this pumping scheme, in which an output power of 3.35 W with slope efficiency of 81.2% was reported [10]. The full power-scaling potential of the laser crystal to produce high output power has not been demonstrated to date. In this work, we address this issue and demonstrate a high-power () CW laser with high output beam quality under 912 nm diode pumping. The results indicate that had times lower thermal lensing than , thus confirming its suitability for power scaling.
Sign up for Photonics Research TOC. Get the latest issue of Photonics Research delivered right to you!Sign up now
2. EXPERIMENTAL SETUP
The schematic energy level diagram of the crystal with the corresponding electron transitions is shown in Fig. 1. It can be seen that the energy difference between the pump and laser photons (912 and 1063 nm, respectively) is much lower in the case of longer-wavelength pumping than with traditional 808 nm pumping. Therefore, pumping at 912 nm can result in a much lower quantum defect as well as higher slope efficiency.
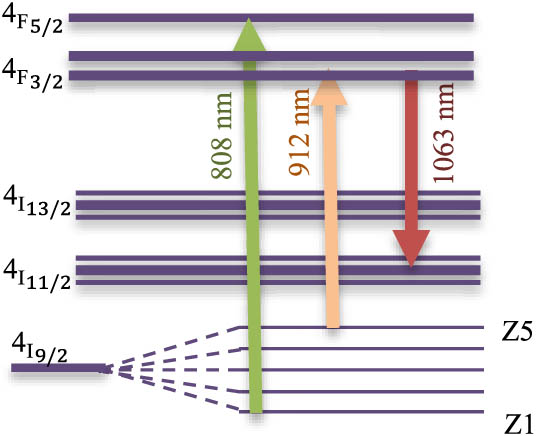
Figure 1.Energy level diagram of .
The gain medium that was used in this work was 1.5 at. % doped a-cut crystal (Castech), which was antireflection coated at the pump and laser wavelengths. The laser crystal was wrapped in indium foil, and the top and bottom surfaces of the crystal were water-cooled at 16°C to remove the heat. The gain medium was pumped at 912 nm by a fiber-coupled laser diode (LD) with 105 μm fiber core diameter and numerical aperture of 0.22. The LD had a spectral width of at half maximum and it was cooled using a thermoelectric cooler. The temperature of the LD was monitored using a built-in thermistor and was set to operate at 25.6°C by a digital temperature controller. Through the use of a couple of focusing lenses, the pump beam was focused into the center of the gain medium with a spot size radius of . Pump absorption in the crystal was measured to be 70%.
The designed laser cavity consisted of three mirrors and is shown in Fig. 2. M1 is a concave mirror (500 mm radius of curvature) and DM is the flat dichroic mirror with high reflectivity at the laser wavelength (1063 nm) and high transmission around 912 nm. The distances L1, L2, and L3 were 90, 355, and 290 mm, respectively. The cavity design was based on ABCD matrix calculations that also considered the effect of thermal lensing. The laser mode size was calculated to be at the center of the crystal, which shows reasonable mode-matching with the pump beam. Furthermore, several output couplers (10%–40%) were tested and the maximum output power was achieved using an output coupler with 25% transmission.
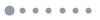
Figure 2.Experimental setup for CW operation.
3. RESULTS AND DISCUSSION
Figure 3(a) represents the measurement results for the laser output power versus the absorbed pump power. The laser had a threshold of about 2.04 W of the absorbed pump power. The maximum output power reached 19.8 W at 33.4 W of the absorbed pump power. The radiation was linearly polarized with E//c-axis. This corresponds to optical-to-optical and slope efficiencies of 59.3% and 62.7%, respectively. This, to the best of our knowledge, is the highest output power achieved to date from an laser with in-band diode pumping at 912 nm, and is almost 6 times higher than the previous maximum reported value [10].
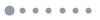
Figure 3.(a) Output power versus pump power (with linear fit) and (b) laser spectrum.
Taking into account the slope efficiency of our system, it is obvious that the highest output power of the laser system was limited by the available pump power and not the thermal effects. The output power can be scaled by using a more powerful pumping source, longer crystal, or multi-pass pumping scheme to compensate for the lower absorption coefficient of at 912 nm as compared to the other pump wavelengths.
Figure 3(b) shows the optical spectrum of the laser output. The peak value was at 1063.3 nm and the linewidth was measured to be around 0.08 nm.
The laser demonstrated an excellent output beam quality and shape. The beam quality factor was measured by a beam profiler and was calculated to be 1.2 and 1.1 in the horizontal and vertical directions, respectively. The measured beam radii and the corresponding values at maximum output power are presented in Fig. 4, where an output beam shape is also displayed.
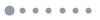
Figure 4.Laser beam quality at 19.8 W of output power. Inset: transverse intensity profile of the laser beam.
Considering the conventional 808 nm diode end pumping, an output power as high as 19.8 W was previously demonstrated [17]. The optical and slope efficiencies were around 50.1% and 58.5%, respectively. However, the output beam quality was not diffraction-limited (). Comparing the present results with the aforementioned data, we obtained the same output power, higher system efficiency, excellent beam quality, lower heating of the crystal, and lower threshold.
There are also a few reports available on high-power lasers with high output beam quality pumped at 880 nm [18,19]. To the best of our knowledge, the highest output power at this pump wavelength was 46 W with slope efficiency as high as 71% [18]. However, this laser used multiple composite crystals in the cavity and a more complicated pumping setup (double-end pumping).
The theoretical upper limit for slope efficiency with 912 nm pumping can be estimated to be around 86%, which is higher than for the 808 and 880 nm pump wavelengths. The lower efficiency of the present work could be attributed to the pump-mode overlap mismatch in the 20 mm long crystal used. Despite the reasonable mode matching at the center of the gain medium, there was a mismatch between the pump and laser mode sizes at the end faces of the laser crystal ( versus , respectively) which reduced the overall efficiency of the laser. The slope efficiency can be maximized by increasing the pump spot size provided that the available pump power can tolerate the increased threshold.
We also investigated the strength of thermal lensing at full power based on a modified ABCD-matrix analysis method [20]. At 33.4 W of absorbed pump power (19.8 W of output power), the focal length of the induced thermal lens was measured to be 120.3 and 112.3 mm in the horizontal and vertical directions, respectively (corresponding to 8.3 and 8.9 diopters).
Comparing these values with the measured thermal lensing of the 19.8 W laser with 808 nm pumping [17], our system offers around 2 times weaker thermal lensing. Recently, we also reported a comprehensive study of thermal lensing effect in the 0.5 at. % crystal pumped at 914 nm [12]. There, a strength of thermal lensing was obtained of up to 6.55 W of the absorbed pump power. Thanks to the calculation of the sensitivity factor [21,22] (), one can calculate the thermal lensing strength at of the absorbed pump power to be above 20 diopters for . Despite similar experimental conditions between this study and the previous work [12], the doping concentrations and pump absorption lengths are different and direct comparison between the obtained data is difficult and can be only approximate. In general, it can be concluded that the present results for the laser show a much weaker thermal lensing effect (around 2 times) under an equal amount of absorbed pump power and similar pumping wavelength. Based on this data, we are confident that the thermal conductivity of the crystal is higher than that of the crystal despite an ongoing debate as to their true values [23–25]. Therefore, due to better thermal performance of the crystal, its output power has the potential to be scaled up to higher levels than with the crystal.
4. CONCLUSION
In summary, using a commercially available pump LD at 912 nm, a 19.8 W laser with low threshold, high efficiency, and excellent beam quality was demonstrated. The maximum power was almost 6 times higher than the previous maximum reported value, with similar pumping wavelength. The strength of thermal lensing at full output power was measured to be an average of 8.6 diopters, indicating a factor of 2 reduction when compared to the laser at 808 nm pumping or laser at 914 nm pumping. The highest output power of the laser system was limited only by the available pump power and not the thermal effects. Compared to the theoretical maximum achievable slope efficiency of 86%, the lower efficiency of this work was attributed to the mismatch between the pump and laser mode sizes. Our results indicate that the 912 nm pumping wavelength is attractive for further power scaling of lasers. High-power operation with excellent beam quality is attractive for mode locking [26–28], wavelength tunability [29], and efficient frequency conversion based, for example, on CW and pulsed optical parametric oscillators [30–33] or second-harmonic generation of visible radiation [34] for pumping of vibronic lasers [35–37].
References
[1] W. Koechner. Solid-State Laser Engineering(2007).
[2] N. Pavel, T. Dascalu, G. Salamu, O. Sandu, A. Leca, V. Lupei. Q-switched Nd lasers pumped directly into the 4F3/2 emitting level. Opt. Commun., 282, 4749-4754(2009).
[3] D. Sangla, M. Castaing, F. Balembois, P. Georges. Highly efficient Nd:YVO4 laser by direct in-band diode pumping at 914 nm. Opt. Lett., 34, 2159-2161(2009).
[4] X. Délen, F. Balembois, O. Musset, P. Georges. Characteristics of laser operation at 1064 nm in Nd:YVO4 under diode pumping at 808 and 914 nm. J. Opt. Soc. Am. B, 28, 52-57(2011).
[5] J. Gao, X. Yu, X. D. Li, F. Chen, K. Zhang, R. P. Yan, J. H. Yu, Y. Z. Wang. 456-nm deep-blue laser generation by intracavity frequency doubling of Nd:GdVO4 under 879-nm diode pumping. Laser Phys., 19, 111-114(2009).
[6] Y.-F. Lü, J. Xia, J.-G. Wang, G.-C. Sun, X.-H. Zhang, A.-F. Zhang, X.-D. Yin, L. Bao, H. Quan. High-efficiency Nd:GdVO4 laser at 1341 nm under 880 nm diode laser pumping into the emitting level. Opt. Commun., 282, 3565-3567(2009).
[7] V. Lupei, N. Pavel, Y. Sato, T. Taira. Highly efficient 1063-nm continuous-wave laser emission in Nd:GdVO4. Opt. Lett., 28, 2366-2368(2003).
[8] H. J. Zhang, L. Zhu, X. L. Meng, Z. H. Yang, C. Q. Wang, W. T. Yu, Y. T. Chow, M. K. Lu. Thermal and laser properties of Nd:YVO4 crystal. Cryst. Res. Technol., 34, 1011-1016(1999).
[9] Y. L. Li, J. Y. Liu, Y. C. Zhang. High efficiency 1341 nm Nd:GdVO4 laser in-band pumped at 912 nm. Laser Phys., 22, 547-549(2012).
[10] J. L. Ma, B. Xiong, L. Guo, P. F. Zhao, L. Zhang, X. C. Lin, J. M. Li, Q. D. Duanmu. Low heat and high efficiency Nd:GdVO4 laser pumped by 913 nm. Laser Phys. Lett., 7, 579-582(2010).
[11] X. Ding, S.-J. Yin, C.-P. Shi, X. Li, B. Li, Q. Sheng, X.-Y. Yu, W.-Q. Wen, J.-Q. Yao. High efficiency 1342 nm Nd:YVO4 laser in-band pumped at 914 nm. Opt. Express, 19, 14315-14320(2011).
[12] T. Waritanant, A. Major. Thermal lensing in Nd:YVO4 laser with in-band pumping at 914 nm. Appl. Phys. B, 122, 135(2016).
[13] R. C. Talukder, Md. Z. E. Halim, T. Waritanant, A. Major. Multiwatt continuous wave Nd:KGW laser with hot-band diode pumping. Opt. Lett., 41, 3810-3812(2016).
[14] T. Waritanant, A. Major. High efficiency passively mode-locked Nd:YVO4 laser with direct in-band pumping at 914 nm. Opt. Express, 24, 12851-12855(2016).
[15] L. Chang, C. Yang, X. J. Yi, Q. K. Ai, L. Y. Chen, M. Chen, G. Li, J. H. Yang, Y. F. Ma. 914 nm LD end-pumped 31.8 W high beam quality E-O Q-switched Nd:YVO4 laser without intracavity polarizer. Laser Phys., 22, 1369-1372(2012).
[16] P. A. Studenikin, A. I. Zagumennyi, Y. D. Zavartsev, P. A. Popov, I. A. Shcherbakov. GdVO4 as a new medium for solid-state lasers: some optical and thermal properties of crystals doped with Cd3+, Tm3+, and Er3+ ions. Quantum Electron., 25, 1162-1165(1995).
[17] J. Kong, D. Y. Tang, S. P. Ng, L. M. Zhao, L. J. Qin, X. L. Meng. High-power diode-end-pumped CW Nd:GdVO4 laser. Opt. Laser Technol., 37, 51-54(2004).
[18] X. Li, X. Yu, F. Chen, R. Yan, M. Luo, J. Yu, D. Chen. Power scaling of directly dual-end-pumped Nd:GdVO4 laser using grown-together composite crystal. Opt. Express, 18, 7407-7414(2010).
[19] H. Lin, J. Guo, P. Gao, H. Yu, X. Liang. High power, diffraction limited picosecond oscillator based on Nd:GdVO4 bulk crystal with σ polarized in-band pumping. Opt. Express, 24, 13957-13962(2016).
[20] H. Mirzaeian, S. Manjooran, A. Major. A simple technique for accurate characterization of thermal lens in solid state lasers. Proc. SPIE, 9288, 928802(2014).
[21] H. Zhao, A. Major. Orthogonally polarized dual-wavelength Yb:KGW laser induced by thermal lensing. Appl. Phys. B, 122, 163(2016).
[22] P. Loiko, S. Manjooran, K. Yumashev, A. Major. Polarization anisotropy of thermal lens in Yb:KY(WO4)2 laser crystal under high-power diode pumping. Appl. Opt., 56, 294-2937(2017).
[23] J. Didierjean, E. Herault, F. Balembois, P. Georges. Thermal conductivity measurements of laser crystals by infrared thermography. Application to Nd:doped crystals. Opt. Express, 16, 8995-9010(2008).
[24] J. Morikawa, C. Leong, T. Hashimoto, T. Ogawa, Y. Urata, S. Wada, M. Higuchi, J. Takahashi. Thermal conductivity/diffusivity of Nd3+ doped GdVO4, YVO4, LuVO4, and Y3Al5O12 by temperature wave analysis. J. Appl. Phys., 103, 063522(2008).
[25] Y. Sato, T. Taira. The studies of thermal conductivity in GdVO4, YVO4, and Y3Al5O12 measured by quasi-one-dimensional flash method. Opt. Express, 14, 10528-10536(2006).
[26] Md. Z. E. Halim, R. C. Talukder, T. Waritanant, A. Major. Passive mode locking of a Nd:KGW laser with hot-band diode pumping. Laser Phys. Lett., 13, 105003(2016).
[27] A. Major, L. Giniunas, N. Langford, A. I. Ferguson, D. Burns, E. Bente, R. Danielius. Saturable Bragg reflector-based continuous-wave mode locking of Yb:KGd(WO4)2 laser. J. Mod. Opt., 49, 787-793(2002).
[28] A. Major, N. Langford, T. Graf, D. Burns, A. I. Ferguson. Diode-pumped passively mode-locked Nd:KGd(WO4)2 laser with 1W average output power. Opt. Lett., 27, 1478-1480(2002).
[29] T. Waritanant, A. Major. Diode-pumped Nd:YVO4 laser with discrete multi-wavelength tunability and high efficiency. Opt. Lett., 42, 1149-1152(2017).
[30] H. Zhao, I. T. Lima, A. Major. Near-infrared properties of periodically poled KTiOPO4 and stoichiometric MgO-doped LiTaO3 crystals for high power optical parametric oscillation with femtosecond pulses. Laser Phys., 20, 1404-1409(2010).
[31] R. Akbari, A. Major. Optical, spectral and phase-matching properties of BIBO, BBO and LBO crystals for optical parametric oscillation in the visible and near-infrared wavelength ranges. Laser Phys., 23, 35401(2013).
[32] S. Manjooran, H. Zhao, I. T. Lima, A. Major. Phase-matching properties of PPKTP, MgO:PPSLT and MgO:PPcLN for ultrafast optical parametric oscillation in the visible and near-infrared ranges with green pump. Laser Phys., 22, 1325-1330(2012).
[33] I. T. Lima, V. Kultavewuti, A. Major. Phasematching properties of congruent MgO-doped and undoped periodically poled LiNbO3 for optical parametric oscillation with ultrafast excitation at 1 μm. Laser Phys., 20, 270-275(2010).
[34] A. Major, D. Sandkuijl, V. Barzda. Efficient frequency doubling of a femtosecond Yb:KGW laser in a BiB3O6 crystal. Opt. Express, 17, 12039-12042(2009).
[35] K. Lamb, D. E. Spence, J. Hong, C. Yelland, W. Sibbett. All-solid-state self-mode-locked Ti:sapphire laser. Opt. Lett., 19, 1864-1866(1994).
[36] S. Ghanbari, R. Akbari, A. Major. Femtosecond Kerr-lens mode-locked Alexandrite laser. Opt. Express, 24, 14836-14840(2016).
[37] S. Ghanbari, A. Major. High power continuous-wave Alexandrite laser with green pump. Laser Phys., 26, 75001(2016).