Abstract
Bismuth (Bi)-doped laser glasses and fiber devices have aroused wide attentions due to their unique potential to work in the new spectral range of 1 to 1.8 μm traditional laser ions, such as rare earth, cannot reach. Current Bi-doped silica glass fibers have to be made by modified chemical vapor deposition at a temperature higher than 2000°C. This unavoidably leads to the tremendous loss of Bi by evaporation, since the temperature is several hundred degrees Celsius higher than the Bi boiling temperature, and, therefore, trace Bi () resides within the final product of silica fiber. So, the gain of such fiber is usually extremely low. One of the solutions is to make the fibers at a temperature much lower than the boiling temperature of Bi. The challenge for this is to find a lower melting point glass, which can stabilize Bi in the near infrared emission center and, meanwhile, does not lose glass transparency during fiber fabrication. None of previously reported Bi-doped multicomponent glasses can meet the prerequisite. Here, we, after hundreds of trials on optimization over glass components, activator content, melting temperature, etc., find a novel Bi-doped gallogermanate glass, which shows good tolerance to thermal impact and can accommodate a higher content of Bi. Consequently, we successfully manufacture the germanate fiber by a rod-in-tube technique at 850°C. The fiber exhibits similar luminescence to the bulk glass, and it shows saturated absorption at 808 nm rather than 980 nm as the incident power becomes higher than 4 W. Amplified spontaneous emissions are observed upon the pumps of either 980 or 1064 nm from germanate fiber.Because of diverse valence states ( to ) and species (monoatomic to cluster ions), the magic element of bismuth (Bi) once doped into solids can emit ultraviolet, visible, near infrared (NIR), or even middle infrared lights[1–20]. For instance as aggregated into polycationic ions, such as , it shows the luminescence of 1–4 μm at room temperature[14]. As doped into different glass systems, it usually shows the luminescence from 1 to 1.6 μm, and it recently was found extending even to 2 μm in silicate glass, also at room temperature[1–3,7,9–14]. The luminescence lies in the spectral range, where rare earth ions cannot fully cover at the same time[21]. The property implies that Bi activated glasses can be the promising gain medium to develop fiber lasers and amplifiers in these new wavelengths. Ever since a continuous wave Bi laser was first demonstrated with Bi-doped silica fiber[4,9,18,19], the studies have become hot topics on exploration of new laser glasses, glass fibers, and fiber lasers.
Current Bi-doped silica fibers are made by modified chemical vapor deposition (MCVD) at the temperature , and such high temperature leads to the severe volatilization of Bi[4,5,9,18,19,22]. Therefore, Bi content is only several tens of parts per million (ppm), and it in turn leads to the very low gain property of such fibers[4,9]. If we could make Bi-doped fibers at a temperature lower than the Bi boiling temperature, a higher content of Bi could reside in the product of fibers. MCVD cannot be applicable to these fibers because of lack of gases of raw materials, such as alkali or alkali earth ions, which can lower the melting point of glass. For this sake, the traditional rod-in-tube method has to be employed. Normally, the technique will first heat the glass preform to the softening temperature and afterward pull it into fibers[23–26]. The process of reheating has been found detrimental to stabilizing the Bi NIR emission center and to simultaneously preserving glass transparency. None of Bi-doped multicomponent glasses previously reported in literature so far can fit the technique of rod-in-tube, since the heating can either extinguish the Bi NIR emission center or devitrify the glass samples. Figure 1 shows the typical scenario of Bi-doped glasses upon a second round of heating. As the inset of Fig. 1 shows, Bi-doped tantalum germanate glass after heat treatment at 750°C for 1 h loses its original reddish brown transparency and becomes devitrified. As a consequence, the luminescence is quenched completely (see curves 1 and 2). So, to make a fiber by the rod-in-tube technique, it is very challenging to find a Bi-doped glass, which can be melted at a much lower temperature, resist thermal impact, and, therefore, allow a high content of Bi.
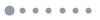
Figure 1.Luminescence spectra of typical Bi-doped tantalum germanate glass (1) before and (2) after being heat treated at 750°C for 1 h upon the excitation of the 808 nm laser diode (LD). Inset shows the change from the original reddish brown transparent sample to the devitrified opaque sample.
In this Letter, we focus on germanate glass. This is because the Ge–O bond strength is weaker than Si–O, and germanate glass can therefore be melted at a temperature much lower than silica glass[1–4]. So, Bi volatilization can be suppressed efficiently, and a higher content of Bi should possibly reside in the glass. It is also because Bi can show stronger NIR luminescence as compared to silica or silicate glasses, and the luminescence bandwidth is typically larger than 300 nm[2–3]. After many times of trial and error, we fortunately found a gallogermanate glass, which can be melted at a lower temperature and can keep glass transparency and luminescence even after heat treatment at a high temperature (see inset of Fig. 2). Further optimization on glass compositions and melting conditions allows us to fabricate the germanate fiber with the rod-in-tube technique. In the end, the spectroscopic properties of such fibers are investigated.
Sign up for Chinese Optics Letters TOC. Get the latest issue of Chinese Optics Letters delivered right to you!Sign up now
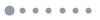
Figure 2.(Color online) Luminescence spectra of gallogermanate glasses doped with different contents (1% to 10%, as labeled) of Bi upon the excitation of the 808 nm LD. Inset shows that the new developed glass keeps the reddish brown transparency though being heated at 600°C for 1 h.
The gallogermanate glasses were prepared by the melting and quenching technique. Glass components were modulated to see the effects on the luminescence properties and to establish the relationship between the composition and refractive index for consequent fiber design. Analytical grade reagents of and , 99.99% pure , , and were selected as the starting materials. Twenty gram batches of each composition were first weighed, homogeneously mixed afterward in an agate mortar, and finally melted in corundum crucibles between in air for 30 min. After that, the melt was poured onto a stainless steel plate and subsequently pressed with another steel plate. The obtained glass samples were cut and polished for consequent optical measurements. For fiber preform, 300 g glass samples were melted in a similar way to the above. A core glass rod with a diameter of about 2 mm was first drawn from the polished cylindrical glass rod in the drawing tower. The cylindrical cladding glass tube was drilled with a hole in the axial direction and mechanically polished. The core glass rod was inserted into the hole of the cladding tube and drawn at 850°C into fiber in the fiber drawing tower.
The refractive indexes of samples were recorded on a prism coupling apparatus (Metricon Model 2010). The NIR emission spectra were measured by a spectrometer (Zolix Omni ), which is equipped with a ZnGaAs photodetector, an SR830 Stanford lock-in amplifier, and an SR540 chopper. Two laser diodes (LD, 808 and 980 nm) and two fiber lasers (980 and 1064 nm) were used as the excitation sources. High temperature was created between by a TAP-02 high-temperature fluorescence accessory (Tian Jin Orient-KOJI instrument Co., Ltd., China). Transient state luminescence was measured by a spectrofluorometer (Edinburgh FLS 920), where a microsecond pulsed xenon flashlamp μF900 with an average power of 60 W was used as the excitation source. The absorption spectra were measured by a Perkin Elmer Lambda 900 UV/Vis/NIR spectrophotometer.
As the inset of Fig. 2 illustrates, all gallogermanate glass samples appear reddish brown, and they can tolerate the thermal impact of the high temperature. All of the samples doped with different Bi contents were melted at 1350°C for 1 h. After being annealed once again, they keep the transparency. As Fig. 3(b) shows, these samples are featured with a strongest absorption peak at and weaker, longer wavelength absorptions extending gradually to 1100 nm. These absorptions are similar to what have been reported for Bi-doped germanate or silicate glasses[1–7,9–17]. As excited into these absorptions, an extremely broad emission can always be detected from 1000 to 1500 nm (see Fig. 2). We have tried to optimize the glass samples in different aspects for consequent fiber fabrication. To improve the luminescence efficiency, we have studied the effects of glass compositions. As Bi content increases gradually from 1 mol% to 5 mol%, we see that Bi NIR emission is enhanced by more than 14 times (see Fig. 2). As the content becomes higher than 5%, the emission starts to be weakened, possibly due to concentration quenching. This can be verified by the dramatic decrease of lifetime from 320 μs for the 1 mol% sample, to 255 μs for the 5 mol% sample, and 137 μs for the 10 mol% sample. We also noticed that the emission peak slightly red shifts for the higher Bi content sample. This should be due to the sample reabsorption. The optimal dopant concentration is therefore 5 mol%, and it is about three orders higher than Bi-doped silica fiber[4,9].
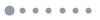
Figure 3.(Color online) (a) Luminescence () and (b) absorption spectra of 5 mol% Bi-doped gallogermanate glasses melted at different temperatures ().
All the glass samples can be melted at 1000°C, but many bubbles stay in the samples. To remove the bubbles, we have tried to increase the temperature, which has been proved efficient. To our surprise, we found that the melting temperature influences the luminescent properties significantly. Figure 3 shows the emission and absorption spectra of the samples melted between 1250°C–1500°C. As the temperature increases from 1250°C to 1350°C, the sample emission is obviously enhanced by more than 50%. A further increase of the melting temperature, however, leads to the decrease of the emission intensity [see Fig. 3(a)]. To understand why this happens, we measured their absorption spectra and found that the concentration of the NIR emission center of Bi actually increases monotonically along with temperature, which should be the reason for the emission quenching. As a consequence, the sample color changes from light pink to purple and to brown [see the inset of Fig. 3(b)]. This implies that the conversion reaction from trivalent Bi oxide to the Bi NIR emission center is thermally, and dynamically promoted by higher temperature. But, at too high a temperature, such as 1500°C, the emission has been quenched severely, and the sample starts to become inhomogeneous [see the inset of Fig. 3(b)]. As we have balanced the tradeoff between luminescence efficiency and Bi distribution within the sample, we eventually choose 1350°C as the optimal melting temperature.
In the long run, thermal degradation of the glass must be considered, especially when the fiber devices work in an extreme condition, and it should shows the long term stability of performance. We, therefore, heated the sample up to 300°C and cooled it back again. The heating and cooling cycle was repeated once to study the degradation behavior. As the environmental temperature rises up, emission begins quenched, and the peak at the same time shifts slightly red (see Fig. 4). This is due to the enhanced interaction between Bi and the host, and the interaction lowers the first excitation state and promotes the nonradiative processes in the meantime. The activation energy was calculated to be 0.014 eV for the quenching[27]. As the sample is cooled, the emission can recover to 94% of the initial intensity. The 6% loss will not increase, and the degradation is reversible in consequent cycling experiments. This implies that though a slight degradation occurs, it does not deteriorate severely. So the glass should be able to work in a reliable way once it is made into fiber and devices.
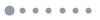
Figure 4.Dependence of emission intensity on temperature during the heating and cooling yoyo experiments.
As we made the bulk glass, machined it into a rod, and drew it in fiber tower, the doped glass does not lose the transparency and NIR luminescence (see Fig. 5). In order to design a cladding fiber, we introduced lanthanum into glass, and its addition has a linear relationship to refractive index . For instance, at 1309 nm, . Similarly, Bi content influences the index linearly, and at 1309 nm, . The measurement error is . Obviously, Bi has a larger effect to the modulation index of the glass than lanthanum. To demonstrate that the glass can be made into fiber, we chose the refractive index difference between the core and cladding as 0.035 and calculated the numerical aperture as 0.35. The composition of the cladding is similar to that of the core, but 2 mol% is introduced to reduce bubbles. The cladding fiber we made is shown in the inset of Fig. 5. The cladding diameter is 125 μm, and core diameter is 10 μm. The propagation loss per unit length was evaluated by the cutback technique, and it is at 1064 nm and at 1310 nm. The losses are partially due to the intrinsic loss of glass host absorption, the Bi absorption, and residual stria or even tiny bubbles. We believe that this can be improved during future bulk glass melting and annealing processes, such as degassing, dehydrating, glass refining, homogenizing, etc. The NIR emission spectra of the fiber sample are the same as the glass sample upon the excitations into 808, 980, and 1064 nm, respectively, in free space. Figure 5 depicts an instance excited by 808 nm, where the emission peaks at with a full width at half-maximum of about 260 nm. This is broader than Bi-doped silica[4].
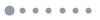
Figure 5.(Color online) Emission spectra of Bi-doped gallogermanate glass (blue line) and fiber (green line), . Inset shows the processes from bulk glass to fiber. Last photo in the inset is the cross section of the fiber.
Absorption saturation was also studied for different excitation schemes. For instance, Fig. 6 shows the emission spectra of Bi-doped gallogermanate glass fiber excited by an 808 or 980 nm LD with different incident powers. Unlike Bi-doped glass samples, fiber samples show saturation of absorption in the case of 808 nm excitation. Initially, as the incident power of the 808 nm laser increases, the emission intensity enhances quickly. However, the enhancement slows down as the power increases to 3.5 from 3.0 W. The emission is weakened as the power is higher than 3.5 W. Interestingly, the scenario differs from the 980 nm pump scheme. Saturation does not appear within the pump power of 5 W [see Fig. 6(b)].
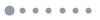
Figure 6.(Color online) Emission spectra of Bi-doped gallogermanate glass fiber excited by (a) 808 nm LD and (b) 980 nm LD with different incident powers.
The amplified spontaneous emission (ASE) spectra of Bi-doped fiber samples were measured by an optical spectrum analyzer (OSA). For direct fiber-to-fiber transmission, fibers were cut in different lengths on both ends and coupled to the fiber pigtails of fiber lasers and OSA by a coaxial butt joint. Two fiber lasers, 450 mW 980 nm and 350 mW 1064 nm, were used as excitation sources, and a 2-m-long Bi-doped silica fiber, which was fabricated by MCVD, was used as the reference. Due to low Bi-doped concentration in the Bi-doped silica fiber, ASE bands are very weak for either 980 or 1064 nm laser pumps. On the contrary, Bi-doped gallogermanate fibers show stronger ASE for these pumps. For instance, as shown in Fig. 7, a 21 cm fiber does not show detectable ASE, while the 33 and 47 cm fibers exhibit stronger ASE, particularly in 1000 to 1300 nm. This implies the the longer Bi-doped germanate fiber leads to the higher ASE band.
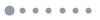
Figure 7.(Color online) ASE spectra of Bi-doped gallogermanate glass fibers with different lengths excited by the 980 nm fiber laser with 450 mW output power. Bi-doped silica glass fiber with 2 m length shows no obvious ASE in the spectral range.
In conclusion, we develop a novel Bi-doped gallogermanate glass, which shows good tolerance to high-temperature impact. This enables the successful fabrication of the first ever, to the best of our knowledge, Bi-doped multicomponent germanate fiber with the technique of rod-in-tube. The fibers show the distinguished ASE with a much shorter length than the Bi-doped silica fiber. This is possibly due to several orders of higher content of Bi and, therefore, higher gain properties in germanate fiber. According to this, we think it is possible to achieve a high-gain Bi-doped fiber with such glass in the future.
References
[1] K. Murata, Y. Fujimoto, T. Kanabe, H. Fujita, M. Nakatsuka. Fusion Eng. Des., 44, 437(1999).
[2] M. Peng, J. Qiu, D. Chen, X. Meng, I. Yang, X. Jiang, C. Zhu. Opt. Lett., 29, 1998(2004).
[3] M. Peng, J. Qiu, D. Chen, X. Meng, C. Zhu. Opt. Lett., 30, 2433(2005).
[4] E. Dianov, V. Dvoyrin, V. Mashinsky, A. Umnikov, M. Yashkov, A. Gur’yanov. Quantum Electron., 35, 1083(2005).
[5] J. Han, L. Li, M. Peng, B. Huang, F. Pan, F. Kang, L. Li, J. Wang, B. Lei. Chem. Mater., 29, 8412(2017).
[6] Y. Zhao, L. Wondraczek, A. Mermet, M. Peng, Q. Zhang, J. Qiu. Opt. Express, 23, 12423(2015).
[7] M. Peng, C. Zollfrank, L. Wondraczek. J. Phys. Cond. Matter., 21, 285106(2009).
[8] L. Su, H. Zhao, H. Li, L. Zheng, G. Ren, J. Xu, W. Ryba-Romanowski, R. Lisiecki, P. Solarz. Opt. Lett., 36, 4551(2011).
[9] I. Razdobreev, L. Bigot. Opt. Mater., 33, 973(2011).
[10] M. Peng, G. Dong, L. Wondraczek, L. Zhang, N. Zhang, J. Qiu. J. Non-Cryst. Solids, 357, 2241(2011).
[11] Z. Song, C. Li, Y. Li, Z. Yang, D. Zhou, Z. Yin, X. Wang, Q. Wang, T. Hau, Z. Zhao, Y. Yang, X. Yu, J. Qiu. Opt. Mater., 35, 61(2012).
[12] F. Kang, M. Peng, D. Lei, Q. Zhang. Chem. Mater., 28, 7807(2016).
[13] X. Guo, H. Li, L. Su, P. Yu, H. Zhao, Q. Wang, J. Liu, J. Xu. Opt. Mater., 34, 675(2012).
[14] R. Cao, M. Peng, L. Wondraczek, J. Qiu. Opt. Express, 20, 2562(2012).
[15] Q. C. Sheng, Q. L. Zhou, D. P. Chen. J. Mater. Chem. C, 1, 3067(2013).
[16] F. Kang, H. Zhang, L. Wondraczek, X. Yang, Y. Zhang, D. Lei, M. Peng. Chem. Mater., 28, 2692(2016).
[17] M. Hughes, R. Gwilliam, K. Homewood, B. Gholipour, D. Hewak, T. Lee, S. Elliott, T. Suzuki, Y. Ohishi, T. Kohoutek, R. Curry. Opt. Express, 21, 8101(2013).
[18] I. Bufetov, M. Melkumov, S. Firstov, K. Riumkin, A. Shubin, V. Khopin, A. Guryanov, E. Dianov. IEEE J. Sel. Top. Quantum Electron., 20, 111(2014).
[19] M. Peng, Q. Zhao, J. Qiu, L. Wondraczek. J. Am. Ceram. Soc., 92, 542(2009).
[20] L. Wang, L. Tan, Y. Yue, M. Peng, J. Qiu. J. Am. Ceram. Soc., 99, 2071(2016).
[21] S. Xu, Z. Yang, T. Liu, W. Zhang, Z. Feng, Q. Zhang, Z. Jiang. Opt. Express, 18, 1249(2010).
[22] M. Ding, S. Wei, Y. Luo, G. Peng. Opt. Lett., 41, 4688(2016).
[23] H. Yu, X. Wang, P. Zhou, H. Xiao, J. Chen. Chin. Opt. Lett., 12, S20604(2014).
[24] W. Li, D. Chen, Q. Zhou, L. Hu. Chin. Opt. Lett., 14, 011402(2016).
[25] J. Liu, L. Ge, L. Feng, H. Jiang, H. Su, T. Zhou, J. Wang, Q. Gao, J. Li. Chin. Opt. Lett., 14, 051404(2016).
[26] Y. Zhao, Z. Wang, H. Yu, X. Xu, J. Xu, X. Xu. Chin. Opt. Lett., 13, 021404(2015).
[27] R. Zhang, H. Lin, Y. Yu, D. Chen, J. Xu, Y. Wang. Laser Photon. Rev., 8, 158(2014).